By chemically tagging genes, sex hormones shape brain
The environment’s influence on gene expression can vary by sex and affect autism’s expression.

Genetic factors undeniably contribute to autism. However, there is mounting evidence that environmental factors may also play a substantial role in the disorder. The environment exerts its influence on DNA through epigenetic modifications — chemical tags on DNA that regulate gene expression without changing the DNA sequence.
Epigenetic modifications come in many forms and can have sex-specific effects. These chemical modifications can silence sex chromosomes, and sex hormones can cause epigenetic changes in the brain. Both of these processes have been implicated in autism.
Understanding the interplay between sex hormones, genes and the social brain is crucial to understanding autism. Most research on this relationship has been done in males, as female hormonal fluctuations complicate an already complex system. The only way to truly understand sex differences in autism is through careful study of both sexes.
There are well-established sex differences in the structure and function of the brain, particularly in regions that support social perception and cognition. Levels of testosterone and estrogen in the womb influence sexual differentiation of the brain, which begins early in embryogenesis.
The chromosomal composition of the developing embryo — males possess a Y chromosome and females have two X chromosomes — partly determines the prenatal hormonal environment. A gene on the Y chromosome causes development of the testes, which produce testosterone. Through epigenetic mechanisms, this sex steroid masculinizes brain and behavior throughout the lifespan.
One hypothesis about the origin of autism, called the ‘extreme male brain’ theory, holds that people with the disorder show exaggerated versions of social, cognitive and behavioral traits that are typical of men1. Researchers have probed testosterone levels among individuals with autism in search of a link but have arrived at mixed results2.
These studies measured testosterone in different tissues from people of varying ages and stages of sexual maturity. Levels of testosterone early in development, when the brain is most plastic, are likely to exert the greatest impact. The few studies that have measured prenatal and perinatal testosterone link high levels to some behaviors associated with autism, but not to a diagnosis3.
X-factor:
Although exposure to high concentrations of prenatal sex hormones may confer an increased risk of autism for boys, the presence of two X chromosomes may act as a protective factor for girls. Around the fourth day of female embryogenesis, one X chromosome in each cell is epigenetically silenced through a process called X inactivation. All cells therefore express only the maternal or the paternal X chromosome.
However, almost one-quarter of the genes on the X chromosome escape inactivation. These genes may serve as a protective factor for females. Similarly, in the days leading up to X inactivation, females may be exposed to a double dose of X chromosome genes, many of which are involved in brain development.
The mechanism that determines which X chromosome is inactivated is under investigation. Some evidence suggests that this process may not be random, and that one X chromosome is more likely to be inactivated in particular tissues. There is also evidence that an X chromosome with mutations may be preferentially silenced.
Even if the selection process is perfectly random, a girl with a mutation on one X chromosome will only express that chromosome in half of her cells. By contrast, a boy with a mutation on his single X chromosome will express it in every cell.
Just as X inactivation can silence whole chromosomes, another epigenetic mechanism can silence specific genes on a chromosome. In a normal phenomenon called imprinting, either the maternal or paternal gene contains an epigenetic mark that silences it.
Disorders occur when the gene from one parent is naturally silenced, and the gene from the other parent is mutated, stamping out expression of that gene altogether. Dysfunction in the imprinting process can also occur, leading to too much expression of a normally imprinted gene. There is some evidence that silencing of the maternal X chromosome may increase the risk of autism4.
Social hormones:
Hormones such as oxytocin and vasopressin also play an important role in shaping sex differences in the brain5. Both of these hormones have been implicated in social behaviors ranging from maternal bonding to play. Both have also been tied to autism6.
Oxytocin and vasopressin are susceptible to environmental influences, early-life experiences and epigenetic changes. For example, the presence of other hormones can influence both the synthesis and binding of these molecules. Vasopressin synthesis is testosterone dependent, whereas changes in oxytocin binding are associated with estrogen levels. The interaction between these social hormones and sex steroids is bi-directional and dynamic.
Exposure to stress — particularly psychological or social stress early in life — also affects social behavior through interactions between oxytocin, vasopressin and the body’s stress response system. Oxytocin levels naturally increase during stress, and administering oxytocin reduces this reaction7.
Intriguingly, social behavior itself can act as an environmental influence. The oxytocin and vasopressin systems interact with neural regions involved in processing information from the environment and are sensitive to socio-environmental context. Both hormones affect the brain and behavioral response to social interaction in a sex-specific way8.
Most studies examining the role of oxytocin and vasopressin in people measure levels of the hormones in the blood and other bodily fluids, or test the effects of synthetic administration. However, the actions of these hormones depend on the activity of their receptors, which have typically not been considered. As a result, these studies have yielded mixed results. For example, a study published earlier this year found that genetic variability in the oxytocin receptor has sex-specific effects on the response to intranasal oxytocin9.
Looking forward:
The presence of oxytocin itself can decrease the expression of its receptor, particularly with continued exposure10. We suspect an epigenetic process called DNA methylation, which silences genes, regulates this negative feedback loop. As a group, women show more oxytocin receptor methylation than do men11. This suggests that they somehow compensate for the effects of methylation, or are more tolerant of hormonal fluctuations or disruptions than men are.
In boys with autism, however, levels of oxytocin receptor methylation are higher in both the brain and blood compared with typically developing boys12. We suspect oxytocin levels in utero influence the developing oxytocin system, and that maternal levels of oxytocin ultimately shape a child’s epigenetic profile. Disrupting the natural balance of oxytocin by using a synthetic version, called Pitocin, to induce or augment labor, for instance, may alter the child’s methylation state.
Epigenetic factors can have subtle but significant effects. In studies examining their influences on behavior, we must carefully consider and control for age, sexual maturity, the type of tissue being studied and other variables. We believe many of the seemingly contradictory results in the literature today reflect our lack of understanding of the intricacies within these systems.
Ultimately, uncovering the mechanisms driving the sex discrepancy in autism will go hand in hand with understanding the neurobiology of the disorder.
Jessica J. Connelly is assistant professor of psychology at the University of Virginia in Charlottesville. Meghan H. Puglia is a graduate student in her lab.
- Baron-Cohen S. Trends in Cognitive Sciences. 6, 248-254 (2002) PubMed
- Schaafsma S.M. and D.W. Pfaff Front. Neuroendocrinol. 35, 255-271 (2014) PubMed
- Whitehouse A.J. et al. J. Neurodev. Disord. 4, 25 (2012) PubMed
- Skuse D.H. Pediatr. Res. 47, 9-16 (2000) PubMed
- Dumais K.M. and A.H. Veenema Front. Neuroendocrinol. Epub ahead of print (2015) PubMed
- Carter C.S. Behav. Brain Res. 176, 170-186 (2007) PubMed
- Carter C.S. et al. Prog. Brain Res. 170, 331-336 (2008) PubMed
- Rilling J.K. et al. Psychoneuroendocrinology 39, 237-248 (2014) PubMed
- Feng C. et al. Genes Brain Behav. 14, 516-525 (2015) PubMed
- Phaneuf S. et al. J. Endocrinol. 154, 7-18 (1997) PubMed
- Puglia M.H. et al. Proc. Natl. Acad. Sci. USA 112, 3308-3313 (2015) PubMed
- Gregory S.G. et al. BMC Med. 7, 62 (2009) PubMed
Recommended reading
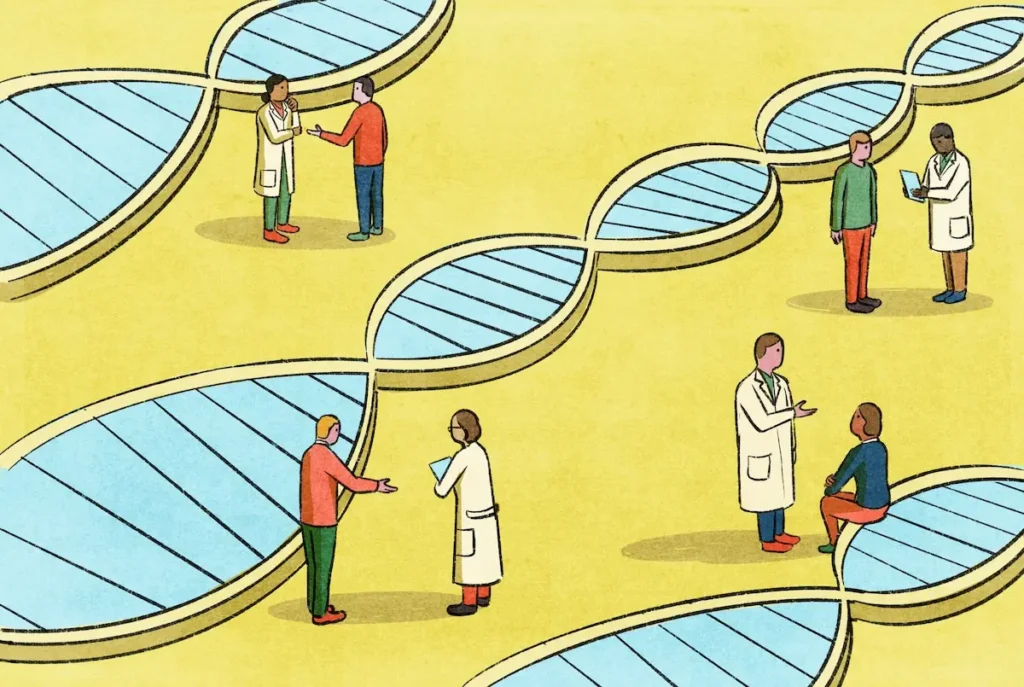
Building an autism research registry: Q&A with Tony Charman
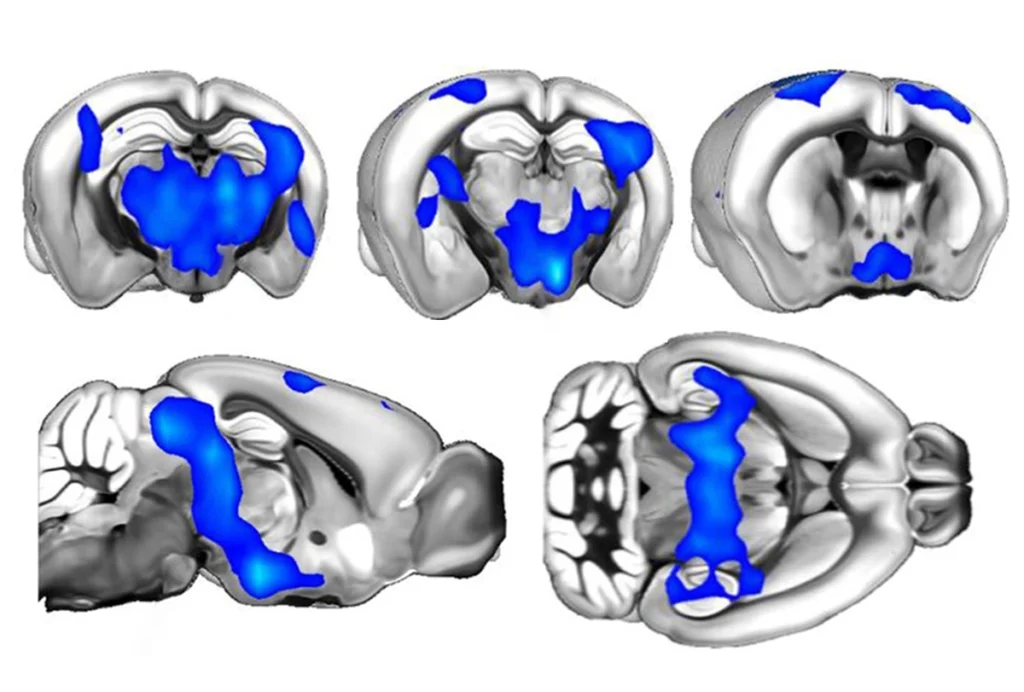
CNTNAP2 variants; trait trajectories; sensory reactivity
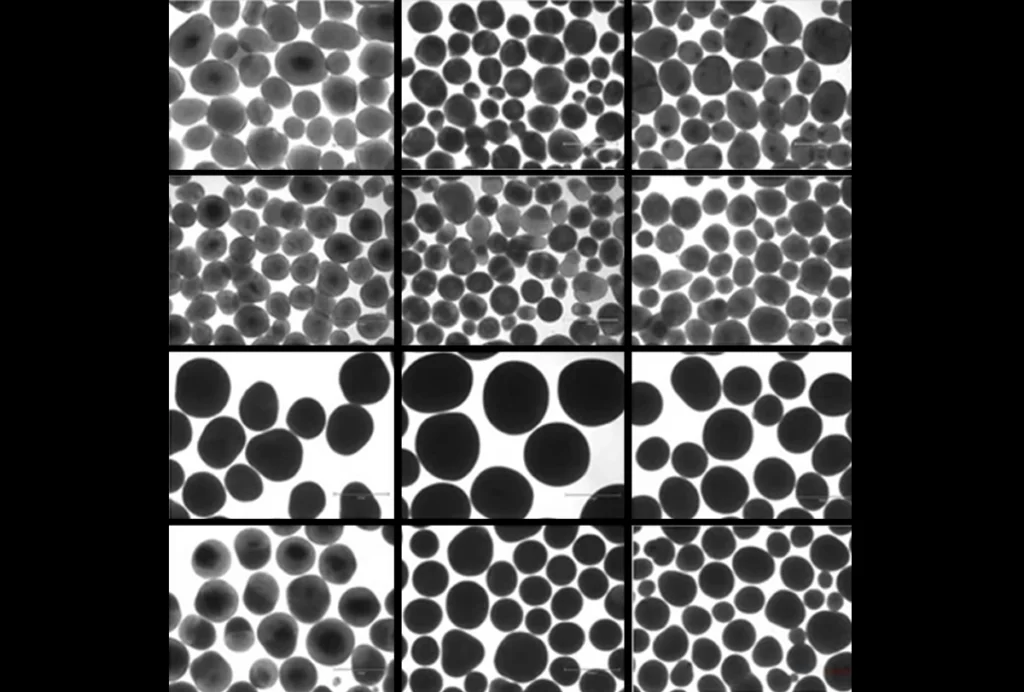
Brain organoid size matches intensity of social problems in autistic people
Explore more from The Transmitter
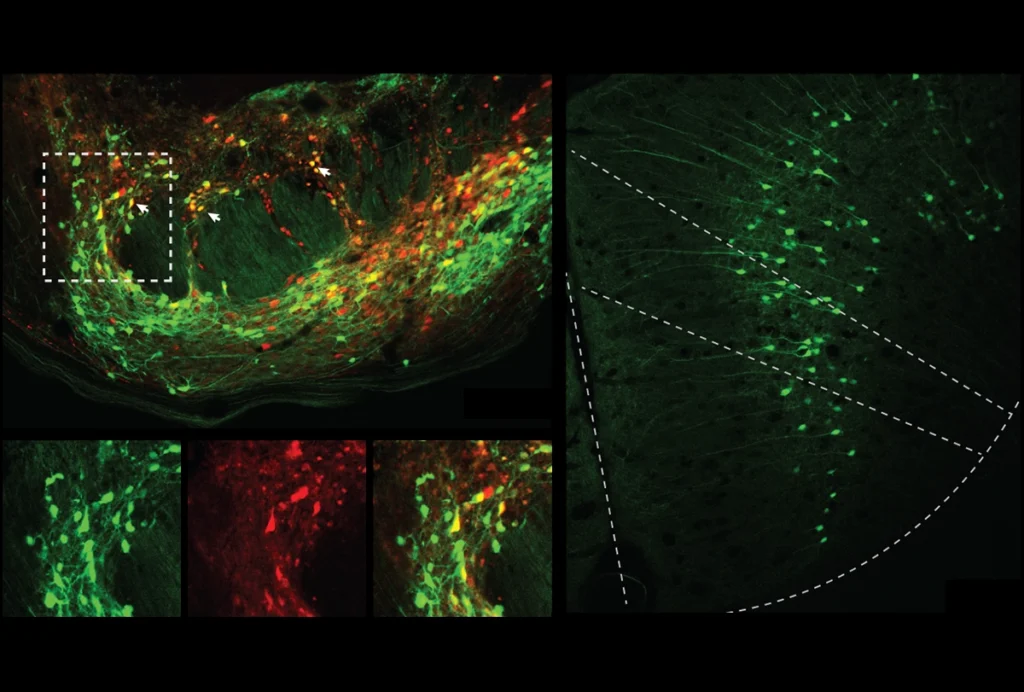
Cerebellar circuit may convert expected pain relief into real thing
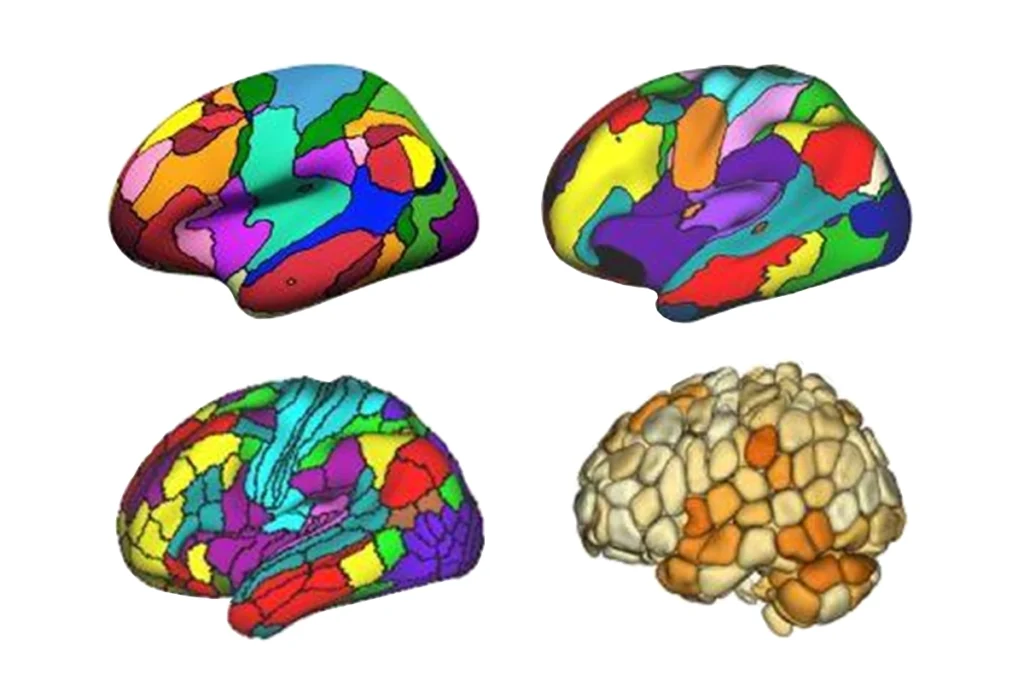