Immature blood vessels may confound baby brain images
A new rat study suggests that baby brain scans should be interpreted with caution. Unlike scans of adult brains, the study says, baby brain scans may show changes in blood flow that do not necessarily reflect the activity of neurons in the region.
It’s one of the hottest areas in autism research: scanning the brains of baby sibs, or the infant siblings of children with autism, in hopes of finding early predictive brain signatures of the disorder.
A new rat study, published 12 March in the Proceedings of the National Academy of Sciences, suggests that these brain scans should be interpreted with caution. Unlike scans of adult brains, the study says, baby brain scans may show changes in blood flow that do not necessarily reflect the activity of neurons in the region.
The issue is specific to imaging techniques that measure blood flow in response to an external stimulus, such as a sound or video. These include functional magnetic resonance imaging (fMRI), which detects magnetic field changes across the brain, andfunctional near-infrared spectroscopy (fNIRS), which uses beams of light to measure activity only in the brain’s outer layers.
The new study found that 12-day-old rats (roughly equivalent to human infancy) show a drop in oxygenated, or red, blood flow when responding to a stimulus — the opposite of what happens in the brains of 80-day-old adult rats1. The juvenile rat brain shows an adult-like response by about 23 days of age (the human equivalent of this age is difficult to pin down).
“In the infant, the whole brain is still developing,” says lead investigator Elizabeth Hillman, associate professor of biomedical engineering and radiology at Columbia University in New York. “The [brain] response isn’t necessarily going to be faithfully reporting neuronal activity in the same way as in the adult brain.”
Out for blood:
The normal adult brain’s response to a stimulus requires a fresh supply of red blood, replete with oxygen. This displaces deoxygenated, or blue, blood from the region. fMRI can track these changes because hemoglobin, the molecule that carries oxygen in blood, is less magnetic when it carries oxygen than when it does not. The blood-flow patterns provide an indirect measure of neuronal activity.
Because of motion artifacts and other practical challenges, fMRI studies of infants and children generally require them to be sleeping or sedated. With fNIRS, in contrast, an awake baby wears a soft cap of noninvasive optical probes that can detect color changes in the blood. This technique also delivers an indirect measure of neuronal activity.
Studies using either technique have revealed wildly conflicting blood-flow patterns in newborn and infant brains. Some reports say that after stimulation, infants show the same increase in red blood that adults do2. But many others have found the inverse response: a sharp increase in blue blood3.
The studies used children of different ages, under different kinds of anesthesia and exposed to different kinds of stimuli, however. “Controlling for these things is particularly difficult,” says Matthew Colonnese, assistant professor of pharmacology and physiology at George Washington University School of Medicine in Washington, D.C., who was not involved in the new study.
In 2008, Colonnese and his colleagues tried to reconcile these studies by adapting an MRI machine for rats. When the researchers gave newborn rats a foot shock, the rats’ brains showed a small and slow influx of red blood that became larger and faster with age4.
Hillman says she wondered whether those results would hold true with a more elaborate technique. She and her colleagues developed a method in which they removed the rat’s skull and scanned the brain’s outer layers with light, similar to the approach in fNIRS.
With this technique, Hillman says, “We can directly observe the dilation and the constriction of blood vessels, we can observe the oxygenation changes, and we can do it all in a very controlled environment.”
In the new study, the researchers tested nearly 100 rats, revealing intriguing blood-flow changes in the brain across development.
When 12-day-old rats were exposed to a foot shock, their brains showed a spike in blue blood and a drop in red — the opposite of what Colonnese’s study found. This happened across the whole brain, which would make sense if the neurons were consuming the oxygen-rich red blood but the nascent blood vessels weren’t replacing it fast enough, the researchers say. Alternatively, it could reflect some kind of global change in neuronal activity, or some combination of changes in neurons and blood vessels.
By 15 to 18 days of age, the rats showed a very short influx of red blood, followed by a surge in blue blood similar to that seen at 12 days. By adulthood, the rats showed the typical, sustained gush of red blood.
“It’s an elegant study,” Colonnese says, and it points to an important practical lesson. In fMRI studies, researchers tend to use algorithms designed to detect responses in adults. “You can’t just port in a model from the adult and expect your imaging data to show neural activity [in infants],” he says.
Baby biomarkers:
The new report may have important implications for autism research.
“We really need to try and work out what this means,” says Sarah Lloyd-Fox, a research fellow in Mark Johnson’s lab at Birkbeck, University of London, who is using fNIRS to study baby sibs. In March, she and her colleagues reported that 4- to 6-month-old baby sibs show less brain activity in response to social sights and sounds than control babies do.
This biomarker may still be real, Lloyd-Fox says. But the rat study suggests it may be a result of differing neuronal activity, or of differences in blood-vessel development, or both. “I absolutely put my hands up and say we don’t know yet.”
One important caveat is that it’s difficult to translate rat ages to those of people.
“We can’t honestly tell you if a [22-day-old] rat is equivalent to a 5-year-old or 5-month-old [person],” Hillman says. Some fMRI studies, she notes, have found children as old as 3 showing the surge in blue blood that never happens in adults.
“I believe it would be dangerous at these early stages to try to define an age range,” Hillman says. Although evidence of the confound in babies is sparse, researchers should be aware of the possibility, she adds.
Not everyone shares these concerns about imaging baby brains.
Charles Nelson, a neuroscience professor at Harvard University who is using fNIRS to study baby sibs, points out that the imaging technique can pick up subtle differences in the response to various stimuli. “If these changes were really due to underlying capillary or blood flow changes, why would they be so sensitive?” he asks.
Blood flow in the infant brain might be particularly sensitive to the level of stimulation, however. In the new study, for example, Hillman showed that strong foot shocks (such as those used in Colonnese’s study) trigger a spike in blood pressure all over the rat’s body, including the brain. This could easily be confused with the surge in red blood that’s typical of an adult neuronal response, she notes.
Hillman plans to follow this up in healthy infants by playing sounds at different volumes and measuring their brain response using fNIRS.
References:
1. Kozberg M.G. et al. Proc. Natl. Acad. Sci. USA 110, 4380-4385 (2013) PubMed
2. Taga G. et al. Proc. Natl. Acad. Sci. USA 100, 10722-10727 (2003) PubMed
3. Yamada H. et al. Neurology 55, 218-223 (2000) PubMed
4. Colonnese M.T. et al. Nat. Neurosci. 11, 72-79 (2008) PubMed
Recommended reading
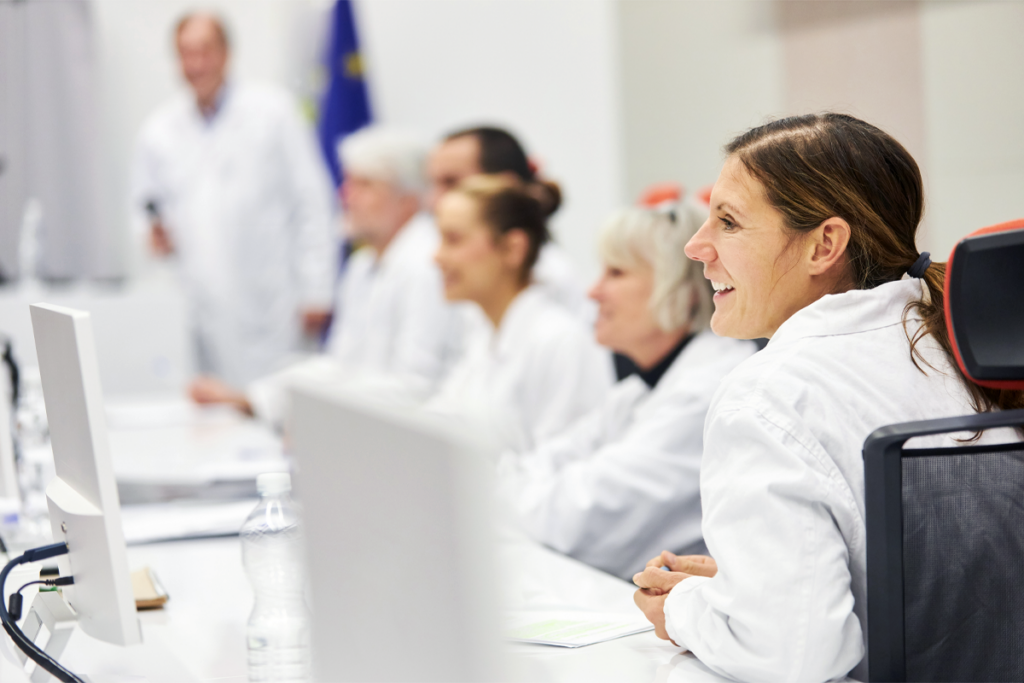
INSAR takes ‘intentional break’ from annual summer webinar series
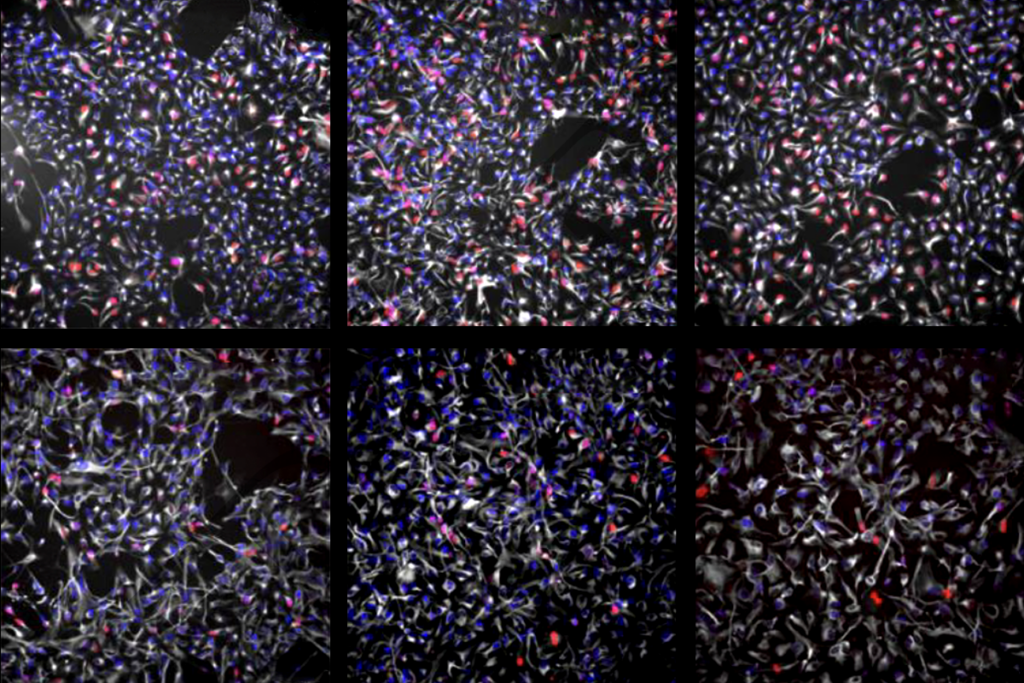
Dosage of X or Y chromosome relates to distinct outcomes; and more
Explore more from The Transmitter
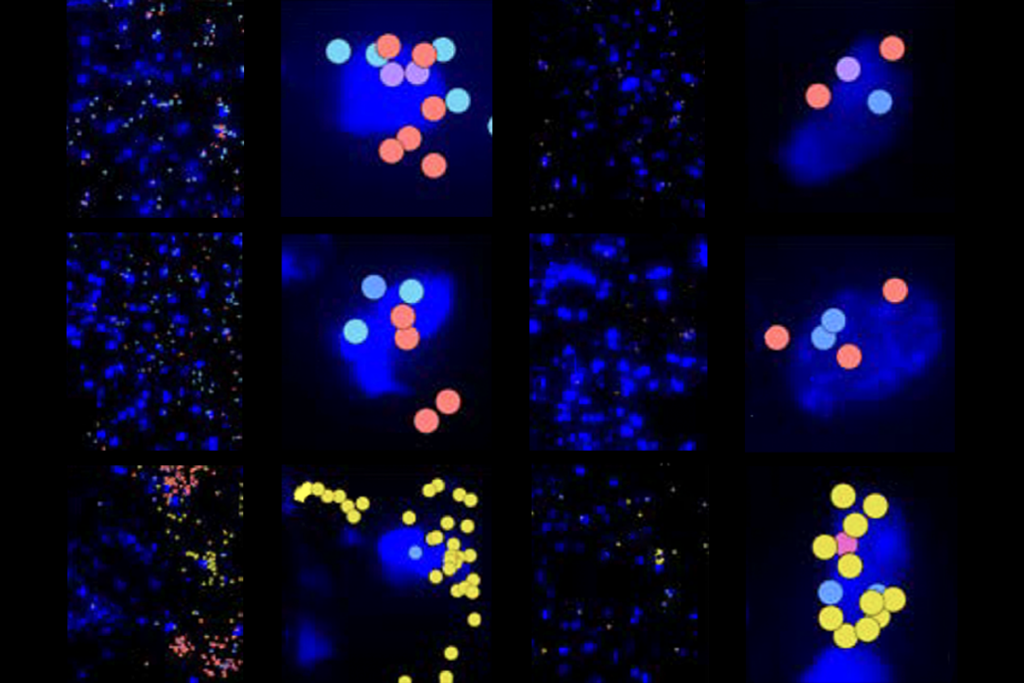
Machine learning spots neural progenitors in adult human brains
Xiao-Jing Wang outlines the future of theoretical neuroscience
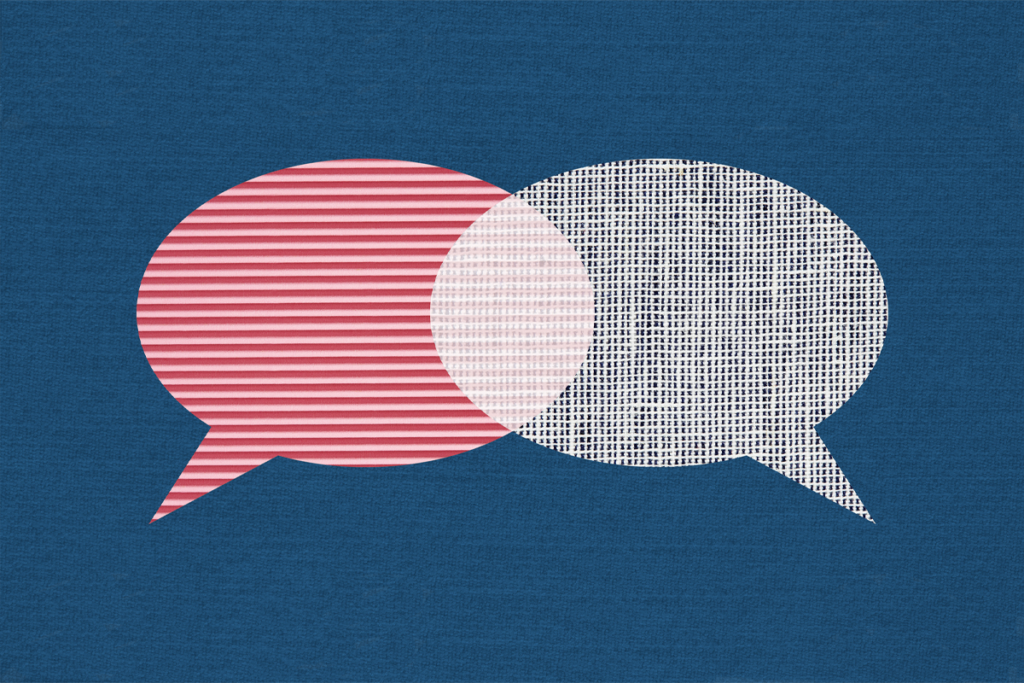