When tracking brain activity, timing can be key
A brain imaging technique called magnetoencephalography characterizes not just what is happening in the brain, but also where and when, making it ideally suited for studying autism.

In a fraction of a second, we experience sensations, form thoughts and interact with our ever-changing world. This speed is critical, as responding too slowly to an attacking lion, a speeding vehicle or a baseball pitcher’s fastball could have catastrophic consequences.
Sluggish brain signaling may also be at the root of neurodevelopmental conditions such as autism. One can imagine how seemingly small lags in processing speech could cascade into significant difficulties during social interactions.
Traditional tools for studying the brain, such as magnetic resonance imaging (MRI), can capture the structure and function of the brain in exquisite detail. But they fail to catch the fleeting fluctuations in neuronal activity that underlie our thoughts, feelings and behavior. Electroencephalography is faster, recording electrical events in the brain that occur in mere fractions of seconds. But the technique cannot consistently separate signals in the brain from those in the muscles covering the skull. It also lacks spatial resolution, offering only coarse insights into the origin of the signals.
A relatively new technique called magnetoencephalography, better known as MEG or simply ‘brainwave scanning,’ characterizes not only what is happening in the brain, but also where and when. Its tracking of purely automatic responses, among other features, also makes it practical for studying people with intellectual disability, and young children. These advantages make it ideally suited for studying complex and diverse behavioral conditions such as autism.
MEG hardware is expensive. There are probably fewer than 200 facilities in the world at this point that house the technology. But its promise cannot be overstated.
Multitasking machine:
MEG records the tiny magnetic fields neuronal activity produces. The machine looks a little bit like a salon hair-dryer, with many hundreds of magnetic field detectors arranged within a helmet that encases the whole head. It must reside in a special room that blocks magnetic fields emanating from the surroundings.
MEG studies have shown that cortical evoked responses — a fleeting form of brain activity that occurs in response to sensory experiences — are atypical in children and adults with autism. For instance, the brain’s response to a ‘beep’ may be delayed by a few thousandths of a second1. This delay may seem insignificant, but it represents a 10 percent slowing compared with what happens in a neurotypical brain.
If every signal traveling through every brain circuit were 10 percent delayed, complex cognitive processes would be significantly slowed, impeding our interactions with the world.
The brain also has baseline rhythms, akin to a heartbeat, that reflect the integrity of the connections between neurons. These rhythms are sensitive indicators of the balance between signals that arouse brain activity and ones that dampen it. They, too, are disrupted in conditions such as autism, and MEG can capture them.
These MEG measurements represent biomarkers that could help to inform clinicians who diagnose autism and researchers who want to study specific subtypes of the condition. MEG may also point to the most appropriate type of intervention for an individual with autism, by highlighting certain signaling lags or atypical rhythms.
The technique might also offer prognostic insights by predicting treatment-related changes in behavioral performance, offering realistic expectations to parents and perhaps guiding the intensity of behavioral interventions.
Moving ahead:
We can consider MEG findings in conjunction with data from diffusion MRI — which measures the integrity of connections in the brain — to investigate the speed of signals between brain regions. We can also combine MEG markers of brain rhythms with magnetic resonance spectroscopy, which measures the chemical contents of the brain, to investigate possible imbalances between excitatory and inhibitory chemical messengers.
In short, instead of competing against other imaging modalities, MEG can augment our arsenal of tools.
As it tracks brain activity, MEG also monitors head motion — and corrects for it. Head motion during an MRI can cause blurring and distort the mathematical analyses of the images. Movements as miniscule as 1 millimeter can render an MRI scan essentially unusable. This limits many imaging studies to the small fraction of children with autism who can understand and follow instructions such as, “Lie still like a statue.”
Because MEG can adjust for at least a few centimeters of head motion, researchers can extend its application to toddlers and individuals with autism who speak few to no words — even those with severe intellectual disability. Not only is the method more inclusive, it also leads to better science in the form of more generalizable conclusions about the entire spectrum.
MEG studies also focus on the brain’s automatic responses, such as its reaction to sound, which do not require the participant to make decisions or plan responses during the experiment.
The ability to include people of all ages from across the spectrum and study the rapid brain changes that underlie interactions with the world makes MEG a powerful tool for autism researchers. Couple this with our strong repository of other brain imaging techniques, and we can gain unprecedented insights into the brain signals that go awry in autism and what they mean for diagnosis and treatment.
Timothy Roberts is Oberkircher Family Chair in Pediatric Radiology and vice-chair of radiology research at the Children’s Hospital of Philadelphia.
References:
- Roberts T.P. et al. Autism Res. 3, 8-18 (2010) PubMed
Recommended reading
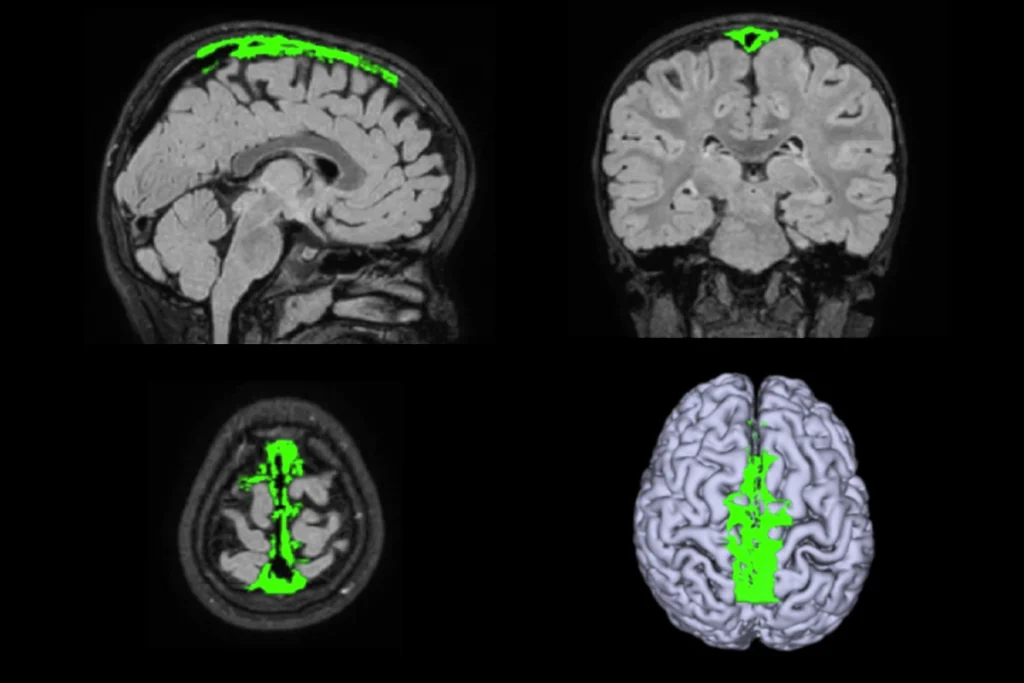
Okur-Chung neurodevelopmental syndrome; excess CSF; autistic girls
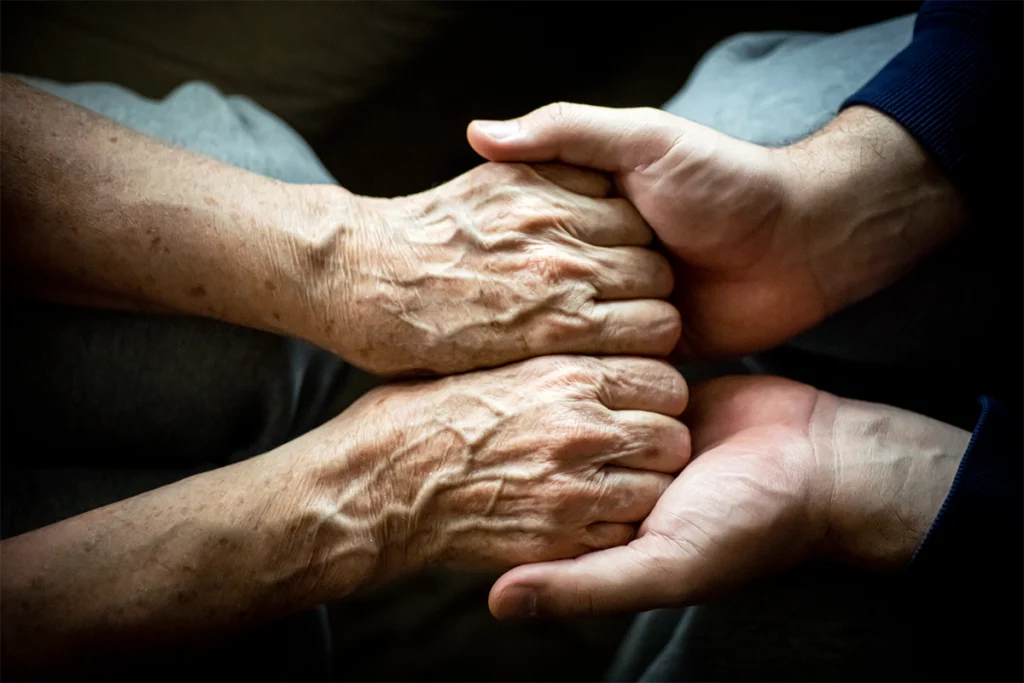
New catalog charts familial ties from autism to 90 other conditions
Explore more from The Transmitter
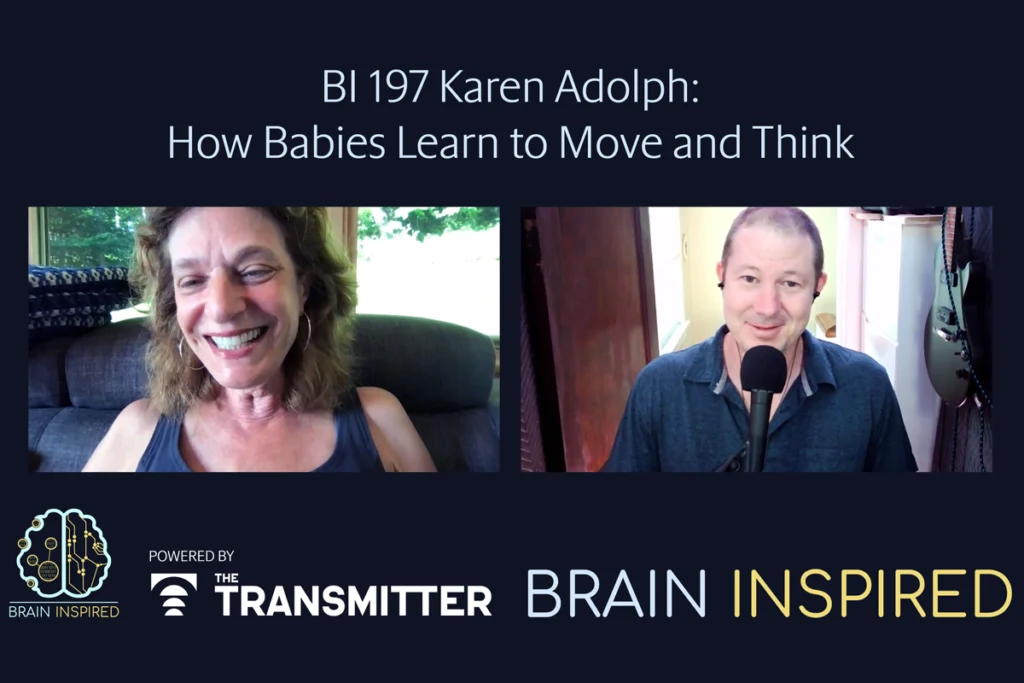
Karen Adolph explains how we develop our ability to move through the world
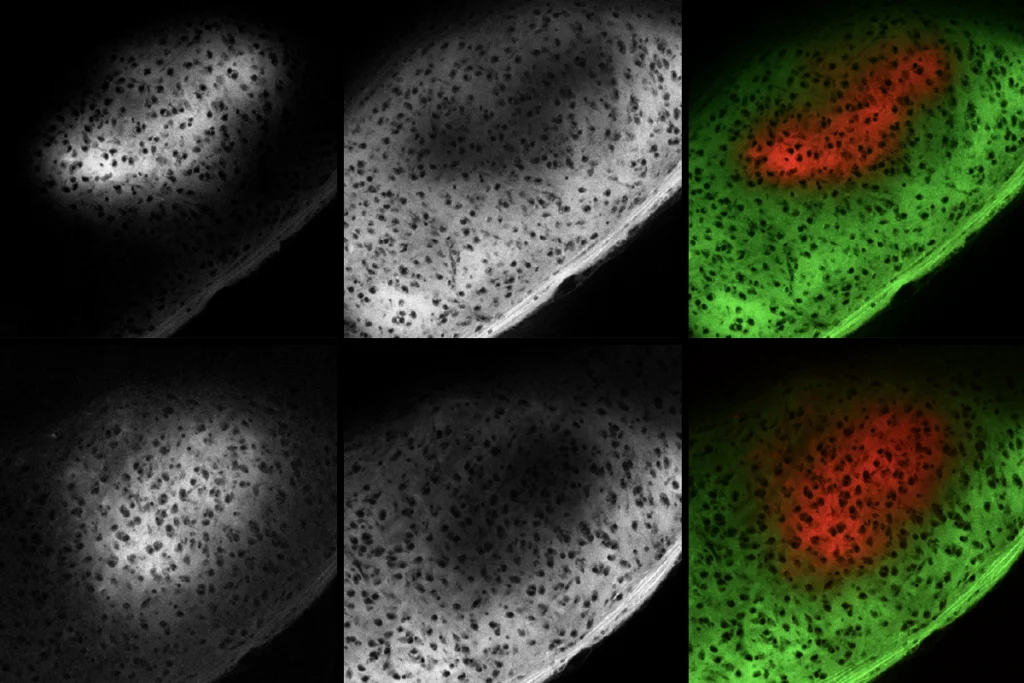
Microglia’s pruning function called into question
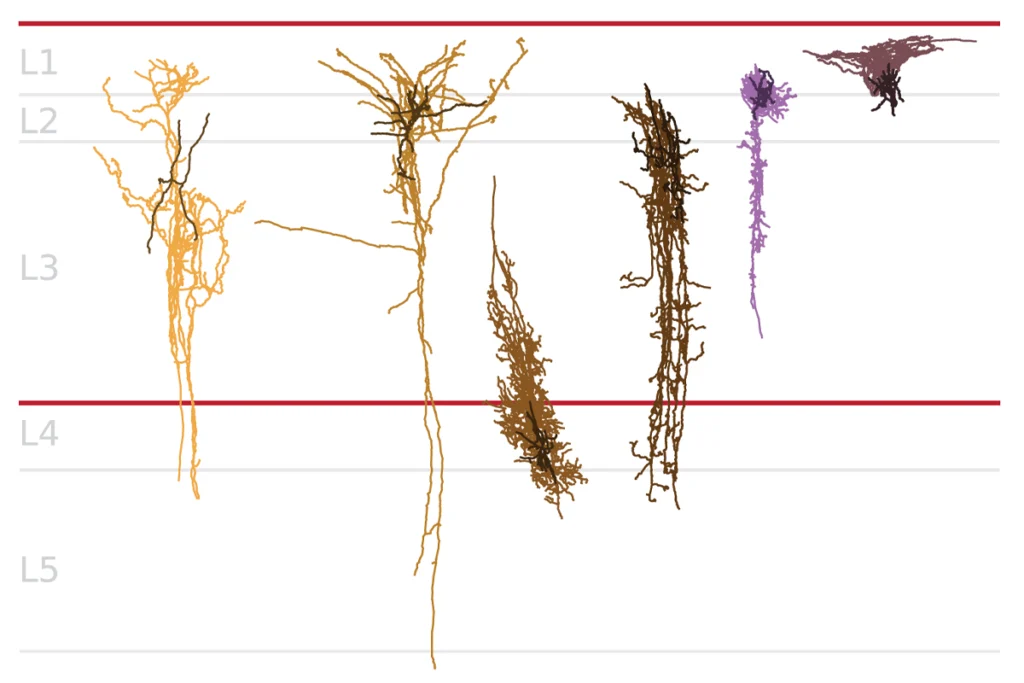