Genome’s ‘dark’ side steps into spotlight of autism research
RNA segments that control when and where genes are expressed may be involved in autism.
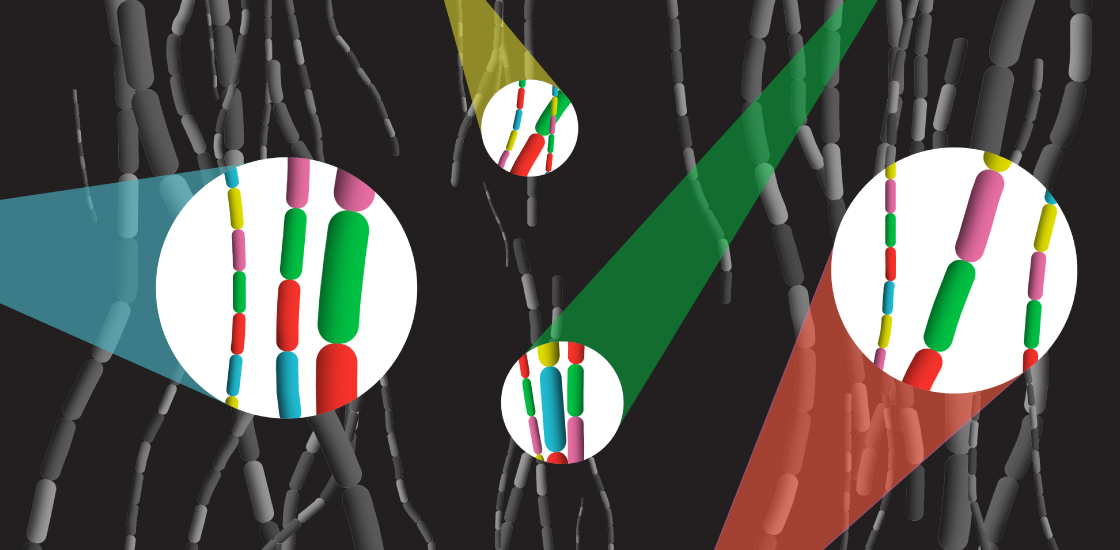
Given that genes make up a paltry 2 percent of the genome, they’ve received a disproportionate amount of attention from autism researchers. Slowly, however, the other 98 percent of the genome — the so-called ‘dark matter’ — is emerging from the shadows.
Once considered nonfunctional or ‘junk DNA,’ these non-gene regions are now known to contain instructions for making pieces of RNA that fine-tune the activity of genes. The RNA segments control when and where genes are active. Autism researchers have looked at the role of these RNAs for only about a decade, but they already have tantalizing clues that the segments seem to be involved in the condition.
“It’s an important field that hasn’t really been studied very much yet,” says Daniel Campbell, assistant professor of psychiatry at the University of Southern California in Los Angeles.
Evidence so far suggests that some noncoding RNAs are unusually scarce and others unusually abundant in people with autism. A few of the RNAs regulate autism genes or signaling pathways implicated in the condition.
Because of this, noncoding RNAs could also lead to treatments for autism.
“When the cause of a disorder is in the regulation of genes, then it might be a better target for intervention than having to repair a gene,” says Dorret Boomsma, professor of genetics and psychology at Vrije University in Amsterdam.
The long and short of it:
There are two major types of noncoding RNA: short stretches called microRNAs, which are roughly 20 nucleotides in length; and so-called long noncoding RNAs (lncRNAs), which have more than 200 nucleotides.
Both types typically turn genes off, but do so in different ways. microRNAs bind to messenger RNA (mRNA), the template for a protein that’s created from a gene, and either destabilize it or block the machinery that translates it into protein. lncRNAs target mRNAs, but they can also bind and block microRNAs. And they can influence gene expression — by interacting either with proteins that turn genes on or off or with those that control how tightly DNA is packed in the nucleus.
Some noncoding RNAs are more abundant in the brain than in other tissues, and seem to be needed for forming neurons and the connections, or synapses, between them.
Changes to the levels of these RNAs “can have serious consequences for brain development and function,” says Boryana Stamova, associate adjunct professor of neurology at the University of California, Davis.
For example, altered levels of noncoding RNA in the brains of people with autism track with a drop in the expression of genes important for brain signaling, and a rise in the expression of genes in the immune system. Both pathways are implicated in autism.
However, each study generates a different list of noncoding RNAs linked to autism, and few RNAs have consistently been tied to the condition. Some of these inconsistencies could be due to variable methods for detecting noncoding RNAs. Also, noncoding RNA expression patterns vary with age, sex, brain region and even cell type — all factors that could contribute to the inconsistencies.
RNAs, interrupted:
Some preliminary genetic evidence hints at how the levels of noncoding RNAs may be altered in autism.
For example, large deletions or duplications in the genome often overlap with noncoding RNAs. Roughly 40 such mutations with strong ties to autism contain known microRNAs1.
Smaller mutations can also involve noncoding RNAs. A 2009 study pinpointed a stretch of chromosome 5 as a site for common variants linked to autism. Campbell’s team explored this region and found that it encodes a lncRNA called MSNP1AS. MSNP1AS turned out to be unusually abundant in the brains of people with autism who carry common variants in this genetic region2.
Campbell’s team discovered that MSNP1AS turns off a gene called MSN that is involved in brain development. Last year, they reported that excess MSNP1AS decreases the number of signal-receiving branches on cultured neurons3. When the researchers tamped down the levels of MSNP1AS, they found changes in the expression of genes involved in the immune system, protein production and DNA packaging4.
“All three of those pathways have been implicated by people looking at protein-coding genes that are mutated in autism,” Campbell says.
Campbell’s team has also reported that CHD8, one of the strongest autism candidate genes, controls the quantity of noncoding RNAs in a cell5.
Some researchers are comprehensively scanning noncoding regions for mutations linked to autism. They are sequencing the whole genomes of people with autism and their unaffected relatives to find spontaneous mutations. Some of the mutations in noncoding RNAs may turn out to contribute to autism, says Ivan Iossifov, associate professor at Cold Spring Harbor Laboratory in New York. “Once we get more data, this will become a very important focus,” he says.
Special delivery:
Other teams are working out the role of noncoding RNAs in animal models.
For example, researchers have found enhanced levels of AK081227, a lncRNA, in a mouse model of Rett syndrome, a condition related to autism. The researchers found that this lncRNA controls the expression of a receptor for gamma-aminobutyric acid, a chemical messenger implicated in autism.
Another study, published in April, showed that Rett mice have increased levels of two microRNAs that impair neuron formation in utero6. Blocking these microRNAs returns neuron formation to normal.
Studies like these hint that manipulating the RNAs might treat autism — although that strategy is not straightforward.
“You can use specific RNA sequences to overexpress or inhibit microRNAs in a mouse,” says Nikolaos Mellios, assistant professor of neuroscience at the University of New Mexico in Albuquerque. “But this is difficult for the clinic.”
The primary hurdle is delivering the RNAs to the brain, because they typically cannot cross the blood-brain barrier. Even if they could, researchers would need to ensure that the RNAs affect only the intended regions.
There are several clinical trials underway using noncoding RNAs to treat cancer and diabetes. Last year, the U.S. Food and Drug Administration approved an RNA-based treatment for spinal muscular atrophy, which is otherwise fatal. The drug must be injected repeatedly into infants’ spinal fluid, and so is unlikely to be adopted for less severe conditions. Still, the approval supports the idea that RNAs can be used to treat neurological conditions.
References:
- Fregeac J. et al. Neurosci. Biobehav. Rev. 71, 729-738 (2016) PubMed
- Kerin T. et al. Sci. Transl. Med. 4, 128ra40 (2012) PubMed
- DeWitt J.J. et al. Genes (Basel) 7, e76 (2016) PubMed
- DeWitt J.J. et al. Dev. Neurosci. 38, 375-383 (2016) PubMed
- Wilkinson B. et al. Transl. Psychiatry 5, e568 (2015) PubMed
- Mellios N. et al. Mol. Psychiatry Epub ahead of print (2017) PubMed
Recommended reading
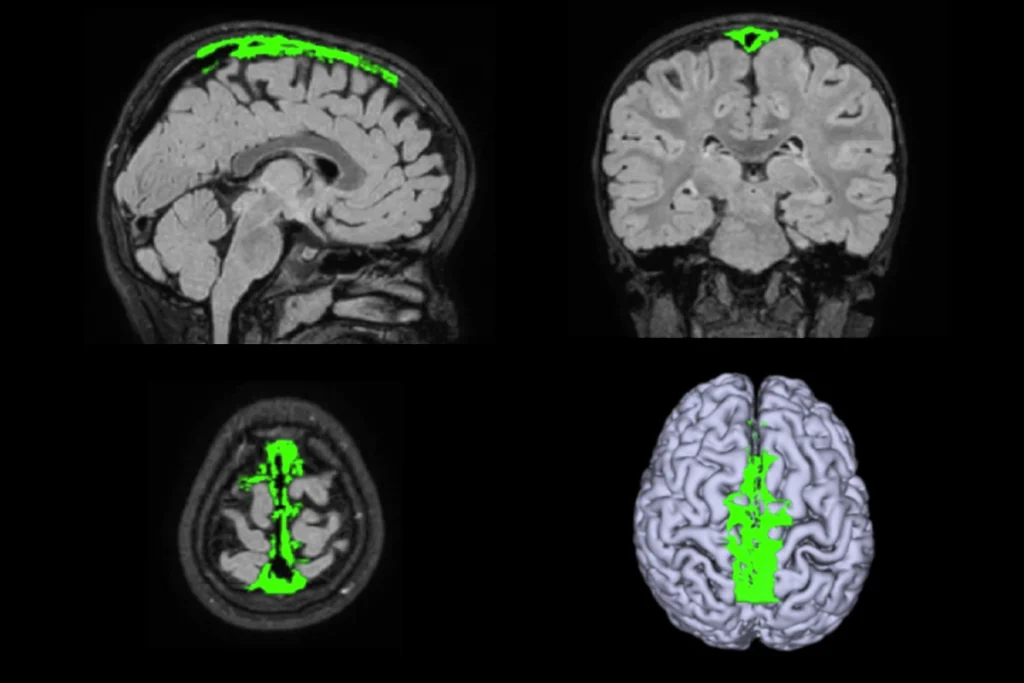
Okur-Chung neurodevelopmental syndrome; excess CSF; autistic girls
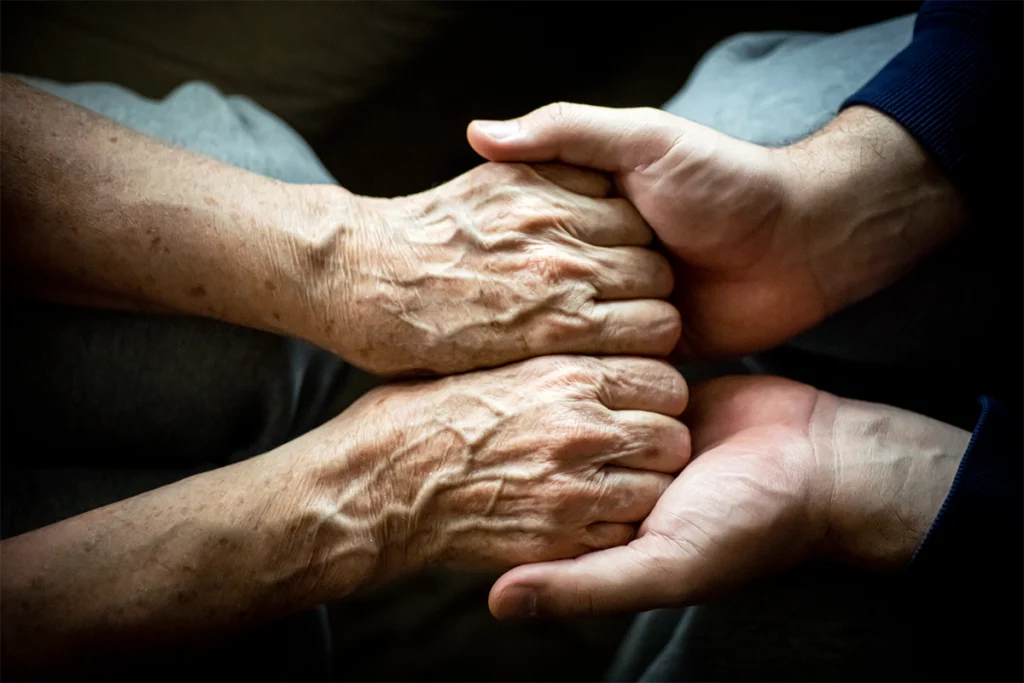
New catalog charts familial ties from autism to 90 other conditions
Explore more from The Transmitter
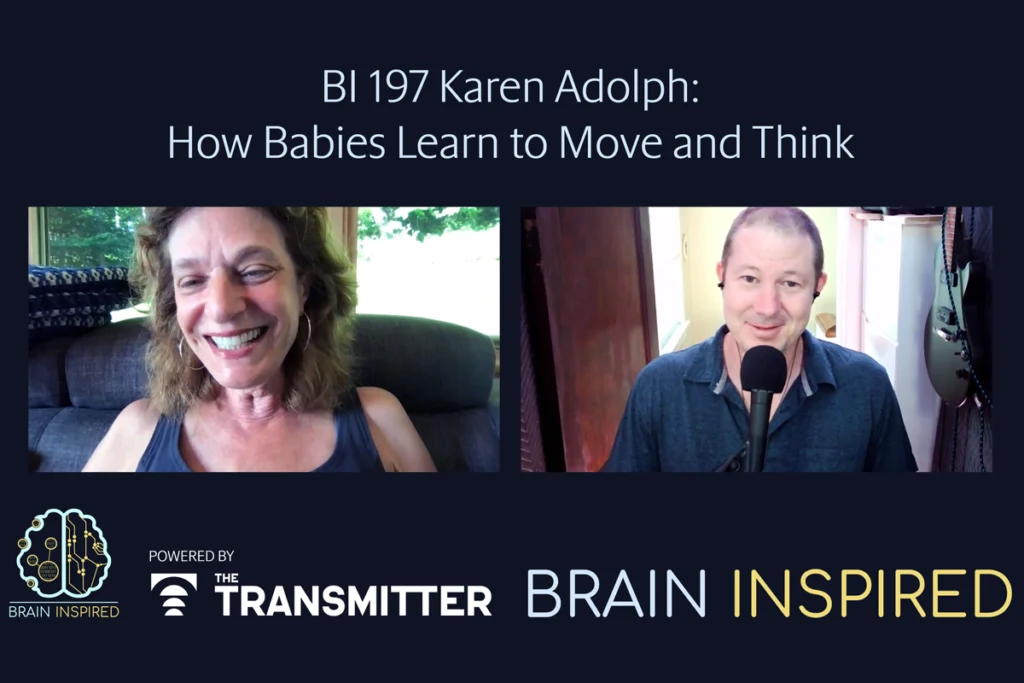
Karen Adolph explains how we develop our ability to move through the world
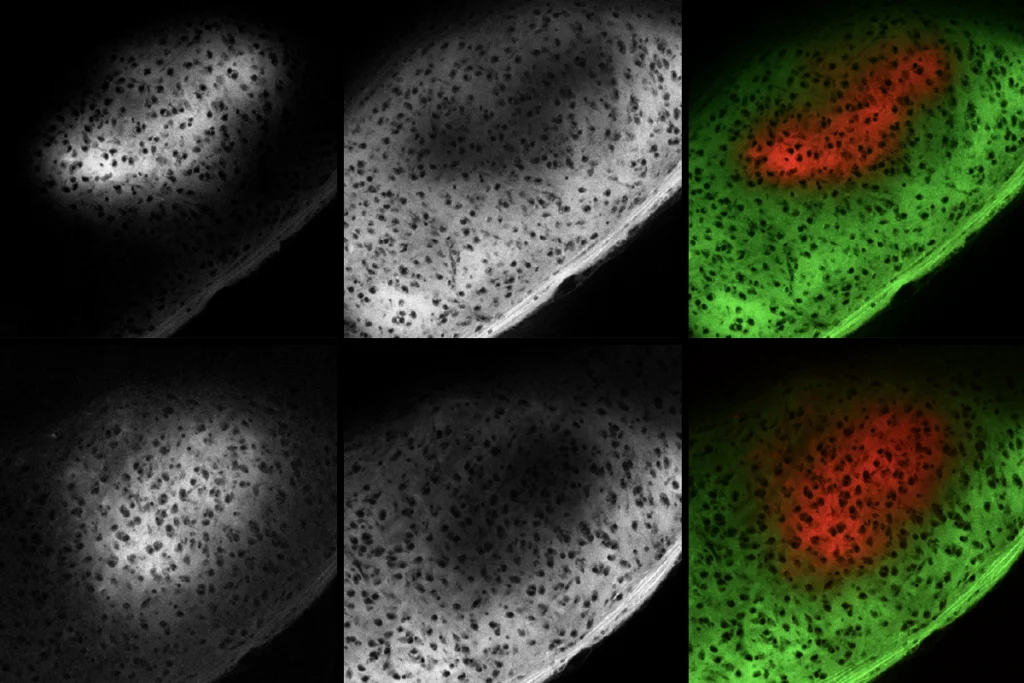
Microglia’s pruning function called into question
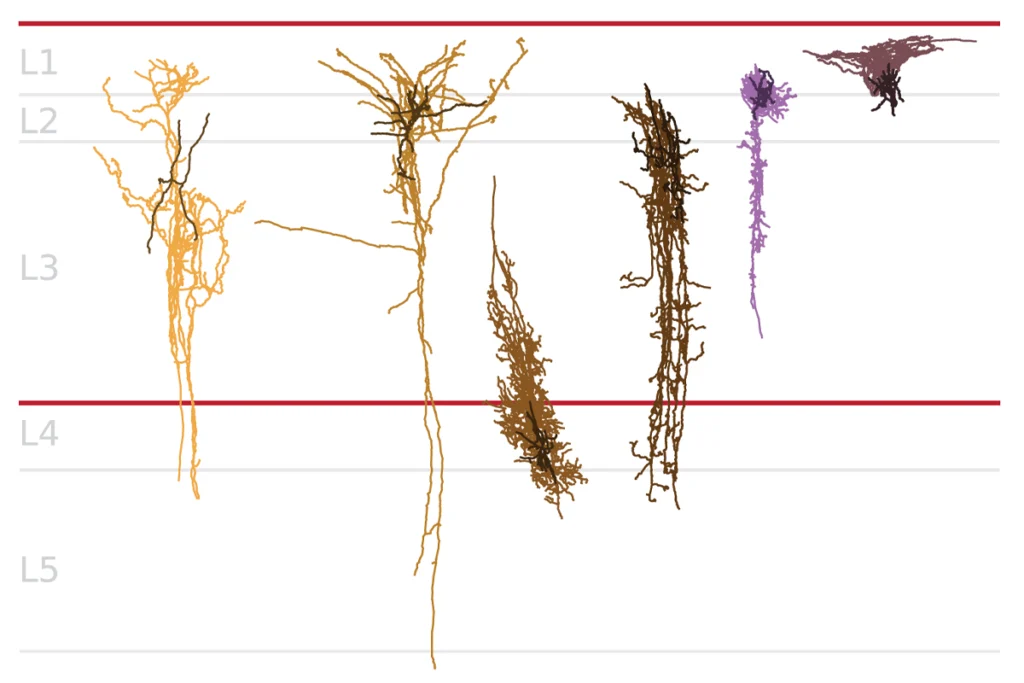