How a ‘pacemaker’ for the brain could ease autism traits
Looking at the brain as a whole suggests that nudging flawed sets of neurons to collaborate better might alleviate autism traits.
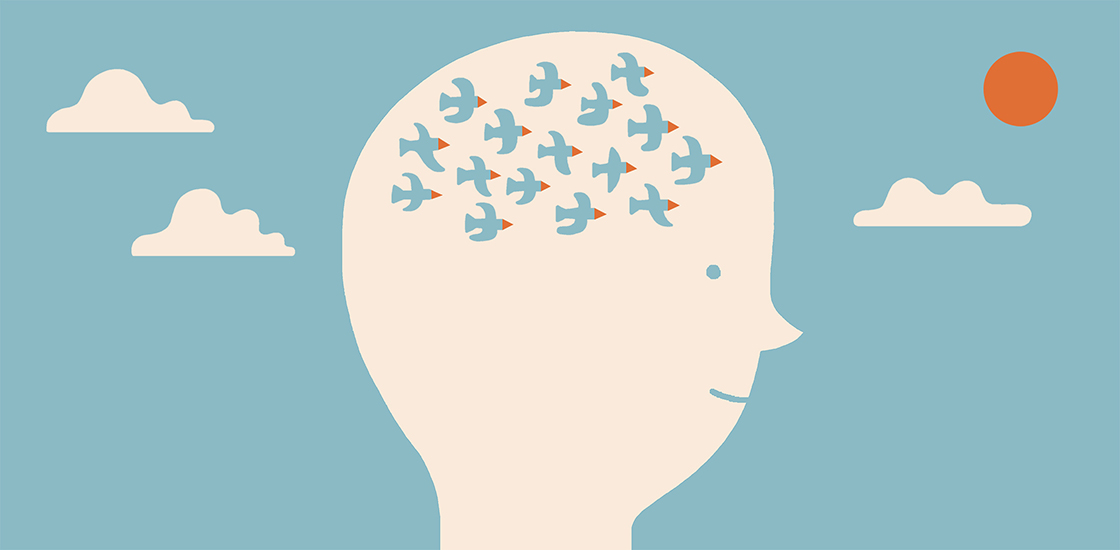
The ability scientists have to manipulate genes has spawned new treatments for cancer and other illnesses. But it has also fostered an obsession with understanding the human body down to its most fundamental building blocks. This granular focus on molecules may at times blind us to the big picture, and to practical solutions.
My colleagues and I have found, in work published over the past two years, the value of considering the brain as a whole rather than dissecting the workings of every last molecule. What we have found suggests that by nudging sets of neurons to collaborate better, we might be able to alleviate autism traits.
There are about 100 billion neurons in the human brain, each of which makes 10,000 connections to other neurons across spaces called synapses. That adds up to about a quadrillion synapses that transmit information across the brain. The brain’s higher-order functions emerge from these pathways, much as the behavior of a flock is more than the sum of the actions of individual birds.
Autism research is often conducted in mice possessing a mutation associated with fragile X syndrome — the most common single-gene cause of autism and intellectual disability. The mutation impairs production of a specific protein found at synapses, which suggests that the neurons themselves might also be dysfunctional.
Until our studies, however, that hypothesis had not been directly tested.
Fragile assumptions:
Most of the existing research involves analyzing brain tissue from dead mice. This can be a powerful and convenient way to elucidate fundamental molecular interactions, but it does not reflect the higher-order functions of the brain, dynamically shifting with new thoughts, attention and information.
My colleagues and I wanted to catch a glimpse of what goes on inside animals’ brains while they are alive and trying to solve everyday problems.
To do so, we employed a systems-level approach and tools that had never before been used in the study of autism: tiny multistranded electrodes implanted in the brain of a mouse to record the electrical activity of dozens of neurons.
Mice that exhibit autism-like features have trouble adjusting their behavior to adapt to changing circumstances. This trait, known as cognitive-behavioral inflexibility, is also a key characteristic of people with fragile X syndrome1,2.
To glimpse the events in the brain during these moments, we tested fragile X mice in a complex task that requires flexibility. As the animals went about the task, we monitored specific neurons called ‘place cells’ in the hippocampus — the brain region that coordinates memory and learning — that help them keep track of where they are3.
The mutant mice learned to do this task as quickly as controls did, but when we changed the task’s details, the mutants had trouble adjusting.
Poor coordination:
In our first systems-level study of this approach, we looked at a mesoscale signature of brain activity: electrical oscillations, which result from millions of synapses activating together. In the mutant mice, those oscillations can appear normal but are abnormally coordinated, especially when the mice are having trouble adjusting their behavior to changing circumstances4.
We expected that individual place cell neurons would also not work properly. But our findings surprised us: Neurons in the mutant mice processed spatial information almost as well as neurons in the controls. In fact, in some ways, they were even more reliable5.
We suspected that what might be abnormal is the higher-level electrical coordination between entire groups of neurons.
The circuits that compute, carry and otherwise process information must synchronize in a precisely timed manner. Otherwise, individual signals will go nowhere or, worse, create misinformation by activating the wrong neurons.
We discovered how to distinguish between oscillation patterns that signal that the hippocampus is in learning mode and those that signal the act of remembering. And we saw that when the mice with the fragile-X-related mutation were struggling to learn new information, their hippocampal circuitry was stuck in recollection mode: It was reporting what the mutant animals had previously learned, instead of the information that was available and necessary to learn6.
This ‘representational inflexibility,’ seen for the first time in an autism mouse model, is presumably due to poorly coordinated activity across the large populations of synapses that generate electrical oscillations and activate neurons.
Setting the pace:
This finding holds promise for a new, and possibly more attainable, approach to treating autism.
Rather than try to repair the molecular dysfunction caused by mutations — a task that scientists have never yet achieved — we could simply focus on nudging the flawed, but perhaps good enough, neurons to better collaborate.
An analogy is the cardiac pacemaker. A heart that is not beating properly often contains damaged or malfunctioning cells. Scientists could conceivably try to repair the cells, but the complexity of cell biology makes that task extremely difficult. Instead, researchers have taken an engineering approach that works extremely well: A pacemaker monitors a person’s heartbeat, delivering an electrical stimulus when the heart slows or skips a beat because electrically active heart cells have failed to coordinate. The heart’s cells do not need to be in perfect condition. As long as they work together, the heart will function.
It’s conceivable that gentle electrical pulses applied to the brain might similarly align errant neuronal signals, perhaps in collaboration with drugs to support the brain’s activity-coordinating mechanisms. These pulses could be delivered directly to the brain as is done with deep brain stimulation or, less invasively, from a cap laid across the scalp, perhaps even at night while the person sleeps.
Applied electrical pulses, an emerging field known as ‘neuromodulation,’ might help coordinate the brain activity of people with autism and ease traits such as behavioral inflexibility.
Several such techniques — transcranial magnetic stimulation, transcutaneous electrical nerve stimulation and transcranial direct current stimulation — are already in use to treat depression, pain and other conditions, with varying success.
It’s early days for neuromodulation, but these minimally invasive procedures might be adaptable to people with autism, enabling them to take in new information and adjust to changing circumstances.
André Fenton is professor of neural science at the Center for Neural Science at New York University.
References:
- Knox A. et al. J. Neurodev. Disord. 4, 2 (2012) PubMed
- Hooper S.R. et al. Neuropsychology 22, 36-47 (2008) PubMed
- Moser M.B. and E.L. Sci. Am. 314, 26-33 (2016) PubMed
- Radwan B. et al. Neurobiol. Dis. 88, 125-138 (2016) PubMed
- Talbot Z.N. et al. Neuron 97, 684-697 (2018) PubMed
- Dvorak D. et al. PLoS Biol. 16, e2003354 (2018) PubMed
Recommended reading
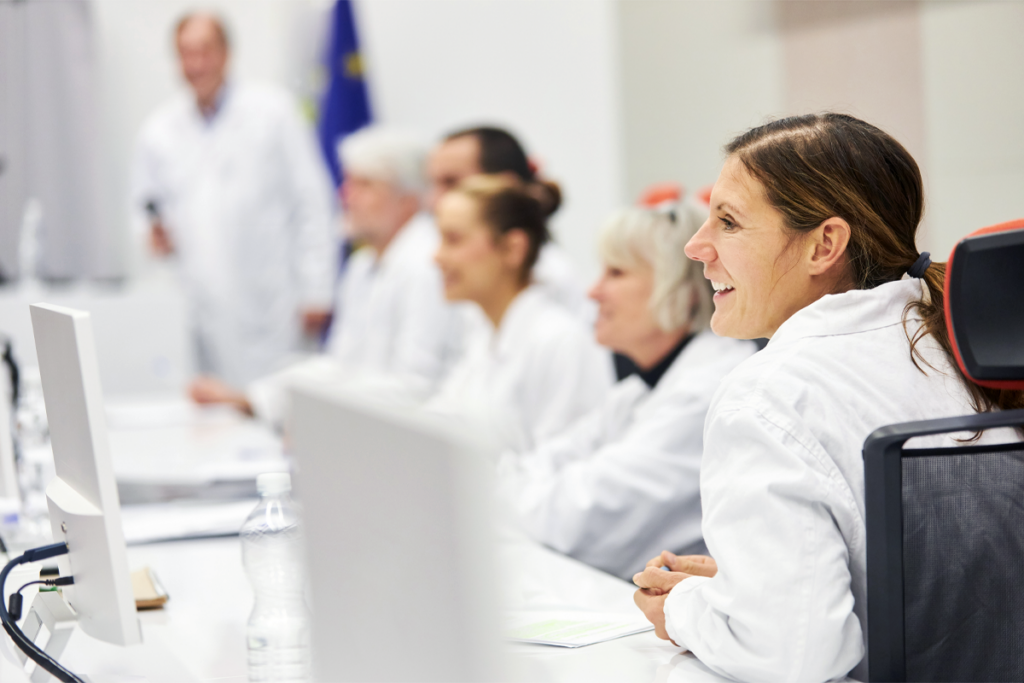
INSAR takes ‘intentional break’ from annual summer webinar series
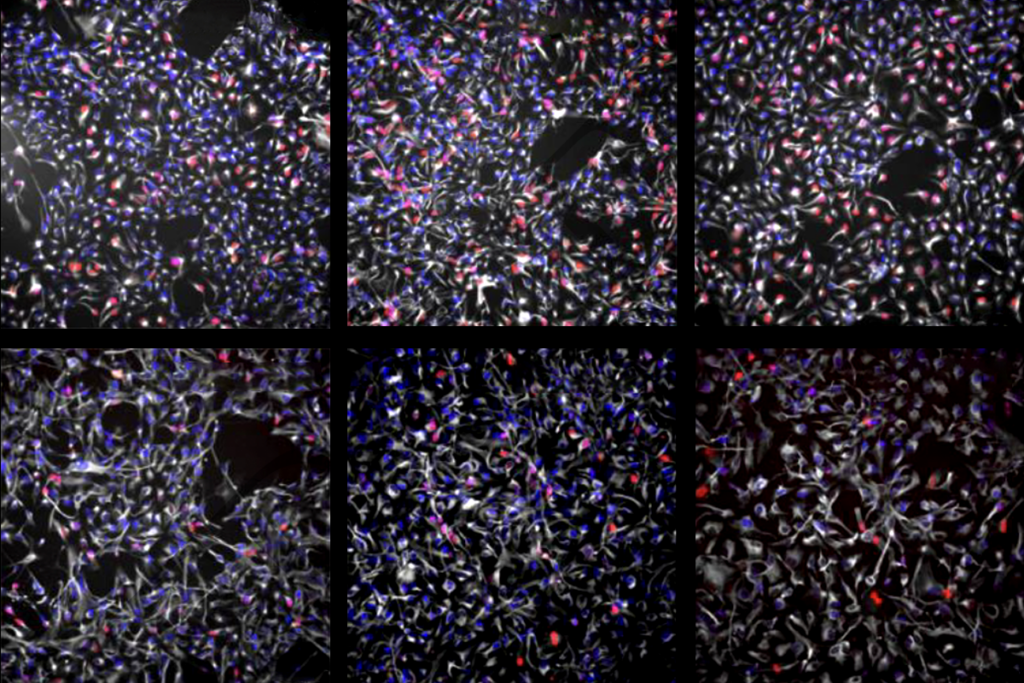
Dosage of X or Y chromosome relates to distinct outcomes; and more
Explore more from The Transmitter
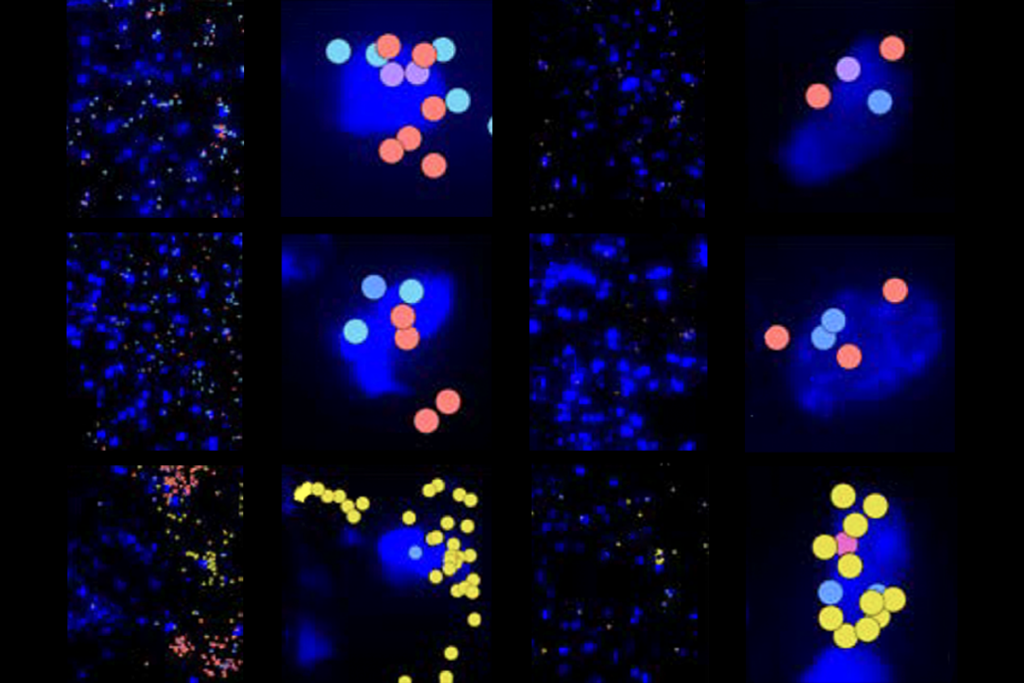
Machine learning spots neural progenitors in adult human brains
Xiao-Jing Wang outlines the future of theoretical neuroscience
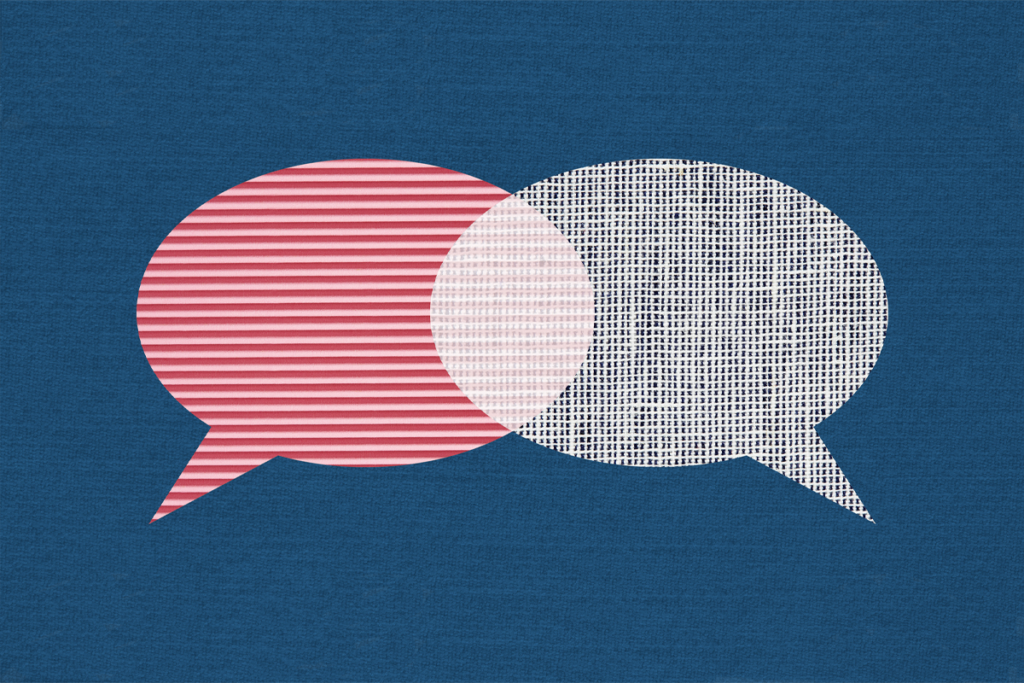