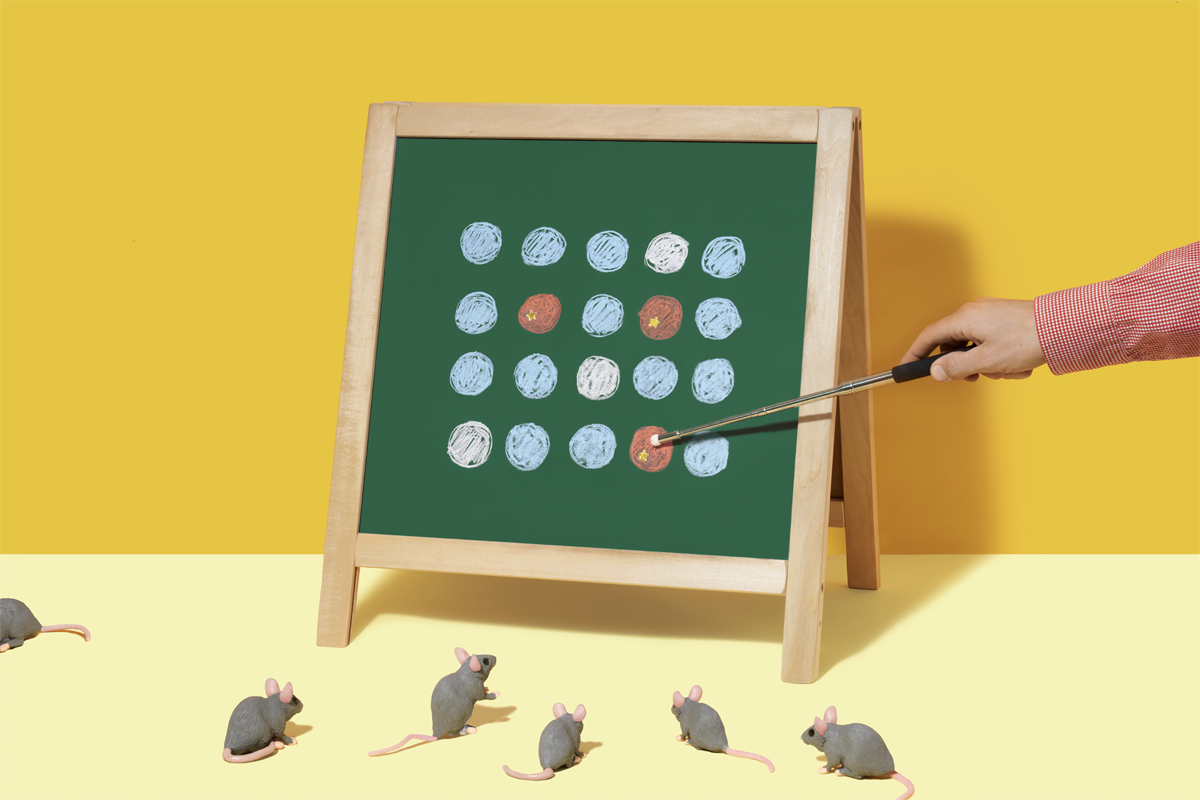
How to teach this paper: ‘Creating a false memory in the hippocampus,’ by Ramirez and Liu et al. (2013)
We’ve known how to implant memories in mouse minds for a decade. Can we implant these ideas in our students?
In the 2010 award-winning film “Inception,” Leonardo DiCaprio’s character and others run around multiple layers of someone’s consciousness, trying to implant an idea in the person’s mind. If you can plant something deep enough, the film suggests, you can make them believe it is their own idea.
The film was billed as science fiction, but three years later, in 2013, researchers actually did this — in a mouse, at least.
The work focused on the hippocampus, along with its closely interconnected structures, long recognized by scientists to hold our dearest memories. If you damage significant portions of just one region of your hippocampus, the dentate gyrus, you’ll lose the ability to form new memories. How these memories are stored, however, is still up for debate. One early but persistent idea posits that enduring changes in our neural circuitry, or “engrams,” may represent the physical traces of specific memories. An engram is sometimes thought of as a group of cells, along with their synaptic weights and connections throughout the brain. In sum, the engram is what DiCaprio’s character would have had to discreetly manipulate in his target.
In 2012, a team in Susumu Tonegawa’s lab at the Massachusetts Institute of Technology (MIT) showed that you could mark the cells of a real memory engram and reactivate them later. Taking that work one step further, Steve Ramirez, Xu Liu and others in Tonegawa’s lab demonstrated the following year that you can implant a memory of something that never even happened. In doing so, they turned science fiction into reality, one tiny foot shock at a time.
Paper overview
Published in Science, Ramirez and Liu’s study is a breath of fresh air, scientifically speaking. The abstract starts with one of the shortest sentences you’ll ever find in a scientific manuscript: “Memories can be unreliable.” The entire paper is extremely readable, and there is no shortage of related papers and review articles that you could give your students to read for additional context.
Pre-reading
Before you dig into the paper, ask your students to watch the TEDx talk by Ramirez and Liu. They use a great set of metaphors to explain the logic of their work and concisely revisit their 2012 paper, which is the basis for this one.
You may also ask students to read more about engrams. The concept has a long, storied history. A 2020 review by Sheena Josselyn at the Hospital for Sick Children and the University of Toronto (and a contributing editor for The Transmitter) and Tonegawa does a wonderful job of explaining the origin of the engram and its scientific growth. Josselyn also has a great talk, available on YouTube, about some of her engram work.
Working through the figures
The paper’s molecular methods section is arguably one of its tricker parts, so it’s worth spending some time on the first figure. It provides methodological validation and includes several ubiquitous features of modern neuroscience papers: a diagram of gene constructs, immunohistochemistry, electrical traces and raster plots, and an overview of the experimental design. Like many memory studies in animals, the work relies on classical fear conditioning: Mice receive a foot shock in a memorable experimental context (basically, arenas of different shapes), remember that shocking experience when they reenter the same context, and freeze out of fear.
Figure 2 presents the key findings of the paper. The team shows that different contexts (“A” or “C”) activate different populations of cells in the dentate gyrus. Figures 2F and 2G demonstrate inception: If you artificially stimulate context-A-related cells in an entirely different context — namely, B — while fear conditioning them (shocking their feet), the mice start to freeze in context A; in other words, they are now afraid of context A despite nothing bad having happened there. The figure also shows a number of controls: The mice don’t freeze in context B without activation of the A cells (either by removing the optogenetic channel or the light), or when any of this is done in a different region of the hippocampus — the CA1 rather than the dentate gyrus. There are more control experiments in this figure and the associated supplementary figures than there are findings — possibly a good point of discussion for a scientist’s continual questioning of “what else could be happening here?”
You could stop here, but Figures 3 and 4 have a few more things to offer. For example, does the fear conditioning from context B conflict with the artificial conditioning from context A?
Using a similar approach to that of Figure 2 but with an additional group of mice that did not have neurons activated during conditioning, the researchers find that mice that had an artificial memory implanted freeze less in context B than mice that didn’t have the memory implanted. In other words, the false memory seems to have interfered with the formation of the genuine memory. If you’re teaching a class with a big focus on behavioral theories, this is a great opportunity to integrate the Rescorla-Wagner model, which helps explain how organisms balance competing needs to learn new things. Furthermore, the amygdala, which receives this fearful information from the hippocampus, doesn’t seem to care whether it’s a genuine or a false memory.
As a final “it’s really happening!” figure, Ramirez, Liu and their colleagues show that creating a false memory is possible not only with simple classical conditioning but also with operant conditioning. They adapt a conditioned place-avoidance task to show that mice actively move away from areas that have a false memory paired with a foot shock, supporting the idea that the mice really do have a false memory and not just some sort of general anxiety response to the researchers putting strange, unfounded ideas into their heads.
Sticky points
Ramirez, Liu and their colleagues use clever, cutting-edge strategies to label and manipulate memory-activated neurons. You can either choose to use this as an opportunity to explain those strategies — especially if you’re teaching a methods-centric course — or summarize them as a means to an end.
Each of the experiments employs one of the many different lock-and-key (“binary expression”) systems that have become ubiquitous in systems neuroscience. Specifically, they use a “Tet-Off” system to control when and where an optogenetic channel (ChR2) and fluorescent reporter (mCherry) are expressed in the brain. Two conditions must be met for ChR2-mCherry to be expressed: The mouse is not fed a special drug (doxycycline) and cells are active, which activates the c-FOS promoter. I strongly recommend “Guide to Research Techniques in Neuroscience” (primarily Chapter 12, accessible through many universities) for a full discussion of these kinds of approaches.
The use of c-FOS is also worth spending some time explaining. It may help to first introduce c-FOS (c for cellular, as opposed to viral) as an immediate early gene that encodes the c-FOS protein, a transcription factor that is expressed when neurons are active. Neuroscience researchers can cleverly track c-FOS (or other things) to mark active cells. Ramirez, Liu and their colleagues measure the c-FOS protein in two different ways, either paired with the Tet-Off system (and therefore with mCherry expression) or by using immunohistochemistry.
The scientists behind the paper
Now assistant professor of psychological and brain sciences at Boston University, lead co-author Steve Ramirez enthuses about this paper as if he just wrote it yesterday.
“The idea of trying to manipulate the concept of a memory cut across all of my favorite Christopher Nolan movies and all of the ideas about what the warpability of memory means … That was the genesis of the idea,” he told me in our conversation about the paper.
The story of how and when the experiments actually happened itself sounds like a movie. Ramirez and Liu were in New York City in 2012, tucked away in an Irish pub to find some drinks and warmth. Into the wee hours of the night, they got talking about follow-up work to their memory reactivation paper. Ramirez had recently read “The Seven Sins of Memory,” by Daniel Schacter, and was mulling over the idea of memory’s malleability. “This does feel like the part in the movie where they totally get the scientists wrong — no one writes on napkins and experimentally plans that way,” Ramirez jokes. But they did. They totally planned this experiment on napkins. A few days later, they met with the engram team they worked with and pitched their idea.
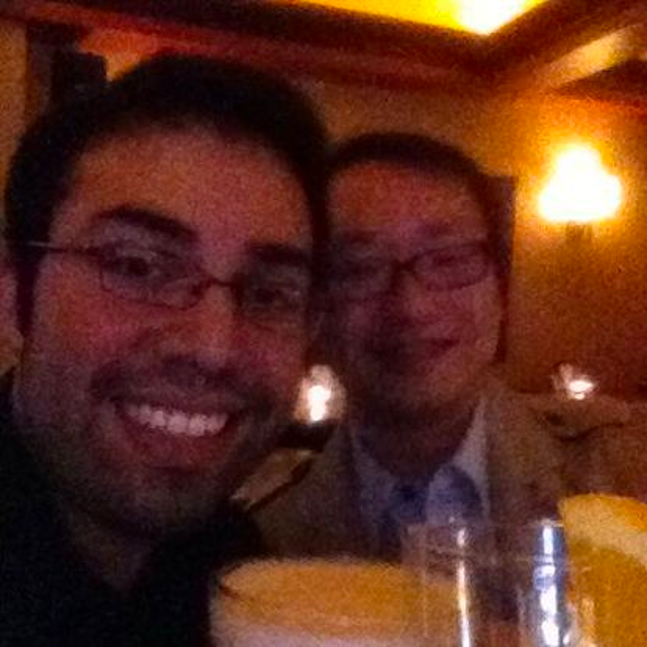
“Within a couple of weeks, we had an answer,” Ramirez says. The day that the experiment worked for the first time was 24 December 2012. Ramirez’s parents were outside waiting to pick him up for Christmas dinner while he was finishing up the experiment and saw the mice freeze with the false memory for the first time. He fired off the data figure in an email to Liu with one attachment: “Inception.pdf”. Subject line? “MERRY FREAKING CHRISTMAS.”
Ramirez loves this experiment not only for the science-fiction element, he says, but also because it’s one of those rare times in science when he cooked up an experiment that actually worked. At the time, it felt like a big gamble — they didn’t know whether straightforward reactivation (their previous work) caused freezing because of memory recall or because they had simply “tickled the hippocampus in the right way,” he recalls. The idea that a few ten thousand cells in the dentate gyrus could reactivate and update a memory is something we might take for granted now, but it was not a given then.
Senior author Tonegawa had a lauded career in immunology before coming to neuroscience, having won the Nobel Prize in 1987 for discovering one of the mechanisms that enable a limited number of genes to produce many different kinds of antibodies. Tonegawa spoke about changing fields in a 2015 interview with Nature, and you can also watch his short talk for the World Economic Forum.
Future lessons
This paper is ripe for extensions into other science-fiction or memory concepts, as well as clinical applications. For example, do modern, experimental treatments of post-traumatic stress disorder (such as the use of ketamine coupled with therapy) work via the same mechanisms? Students might also be invited to think about the power of manipulating memory in a positive way, the focus of Ramirez’s more recent work (e.g., Grella et al. 2022).
Have you taught or studied this paper in the past? What tips can you share with the neuroscience community? Leave a comment below.
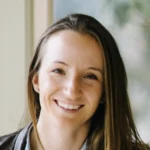
University of California, San Diego
Recommended reading
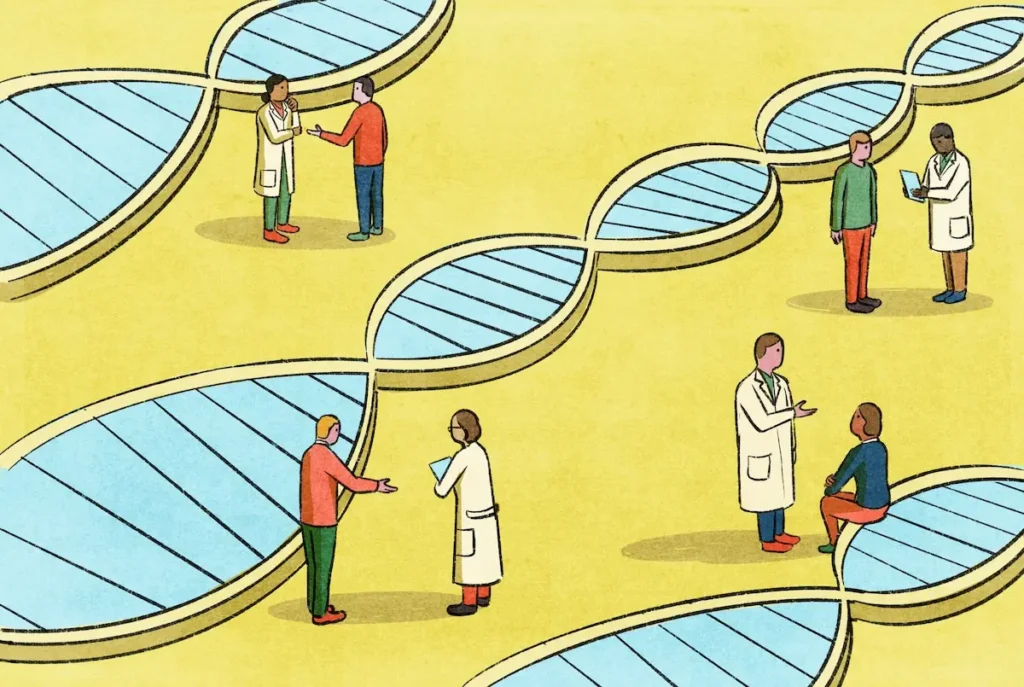
Building an autism research registry: Q&A with Tony Charman
Explore more from The Transmitter
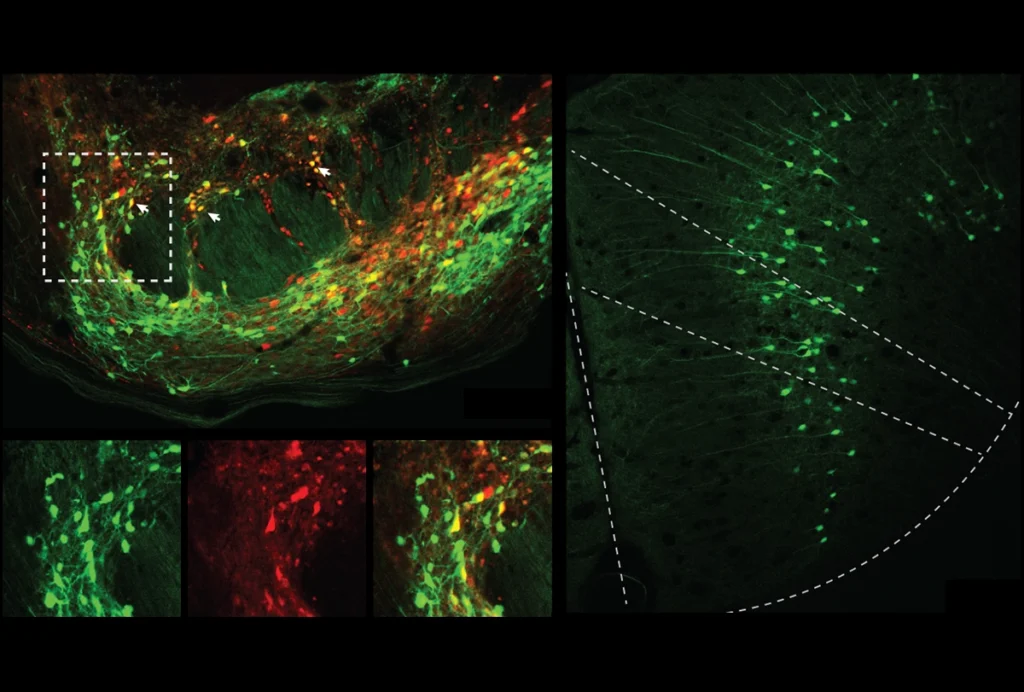
Cerebellar circuit may convert expected pain relief into real thing
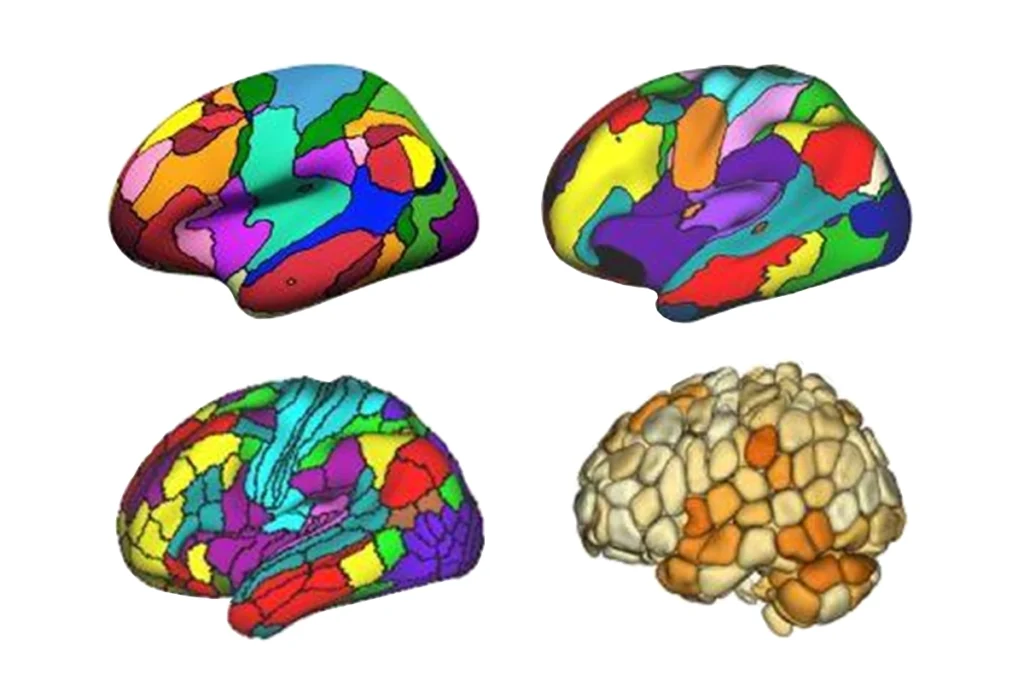