Optogenetics study boosts signal imbalance theory of autism
By zapping mouse brains with blue and yellow light beams, scientists have manipulated the animals’ social behaviors and bolstered a popular theory of what causes autism.
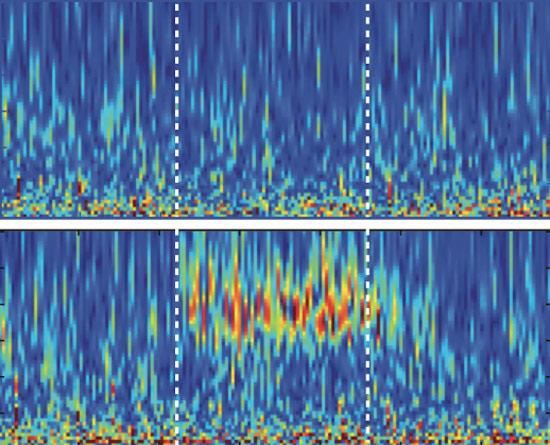
By zapping mouse brains with blue and yellow light beams, scientists have manipulated the animals’ social behaviors, according to a study published 27 July in Nature1. The report bolsters the popular hypothesis that autism stems from an imbalance between excitatory and inhibitory signals in the brain.
Using a technique called optogenetics, the researchers engineered mice to carry light-sensitive proteins in excitatory neurons in the prefrontal cortex, a brain region involved in learning and social behavior. Stimulating these neurons with light raised their firing rates for about a half an hour. During that period, the animals were not interested in sniffing or interacting with other mice, presumably as a result of the increased excitation.
“They were completely uninterested in engaging socially,” says lead investigator Karl Deisseroth, professor of bioengineering and psychiatry at Stanford University in Palo Alto, California. “It was a very profound difference — one of most profound behavioral differences we’ve seen in all our work.”
Deisseroth developed the optogenetic technique in 2004 and has used it to investigate a host of behaviors, ranging from basic movements to anxiety.
The study boosts a theory published in 2003 proposing that autism is caused by an imbalance in the brain: too many excitatory signals and too few inhibitory ones to quiet the clamor2.
Several lines of evidence fit this hypothesis. About one-third of individuals with autism also have seizures, a physical manifestation of runaway brain activity. Many autism candidate genes — such as GABRB3, NLGN3 and SHANK3 — encode proteins that are important for balanced brain signaling. And some imaging studies have shown that individuals with autism have too much activity in frontal areas of the brain.
The theory has been tested in mice carrying mutations that cause a signaling imbalance, notes John Rubenstein, Distinguished Professor of Child Psychiatry at the University of California, San Francisco, and one of the theory’s original proponents. However, with this approach, the animals’ behaviors may also be influenced by any compensatory mechanisms they develop during their lifetime.
For instance, last year Huda Zoghbi‘s team at Baylor College of Medicine in Houston created mice lacking MeCP2 — the gene missing in Rett syndrome — only in neurons that produce GABA, an inhibitory neurotransmitter. These mutant mice produce less GABA than controls, and show abnormal social behaviors and repetitive grooming.
The new study is the first to test the imbalance theory in a short time frame, “where there isn’t a lot of time for compensatory functions to act,” Rubenstein says. “It’s very exciting.”
The researchers relied on new optogenetic technologies to stimulate different types of neurons for up to 29 minutes, about 20 times longer than in previous studies.
In those studies, a barrage of light pulses would travel down a fiber optic cable snaked through a surgical shunt in the animal’s skull. This technique is not ideal for studying social behaviors, however. “When one animal is interacting with another, we didn’t want a fiber optic implant on its head,” Deisseroth says.
The light-sensitive proteins, called opsins, sit in the outer membrane of a neuron. When stimulated with light, they allow ions to flow into the cell, priming it to fire more quickly.
In the new study, Deisseroth’s team designed more stable opsins that, once stimulated, keep the channels primed for long periods of time. The scientists also made opsins that are sensitive to different wavelengths of light.
Using viruses, the researchers inserted blue-sensitive opsins into excitatory cells in the prefrontal cortex of adult mice, and yellow-sensitive opsins into inhibitory cells. That way, blue light would selectively activate excitatory cells, and yellow light, inhibitory cells.
After being exposed to blue light alone, the animals showed a normal range of movements, anxiety and responses to new objects. Unlike typical mice, however, the mutants did not approach new playmates.
Then, using both blue and yellow light, the researchers simultaneously boosted excitatory and inhibitory signals, effectively normalizing the balance of excitation and inhibition, and partially restoring the mutants’ social deficits. When mice exposed to both lights were given the option of exploring a new object or a new mouse, they preferred the latter.
Because of technical limitations associated with yellow opsins, for this part of the experiment, the researchers had to use the older technique of continuous pulsing through the fiber optic cable. Still, the results are encouraging, Deisseroth says.
“This was a very stringent test of the imbalance hypothesis,” Deisseroth says. The boost in inhibitory activity, even though it is an abrupt and abnormal change to the brain, levels the balance of excitation to inhibition. “It actually made things a little better.”
The opening salvo:
The new study also shed light on the largely mysterious link between autism and high-frequency brain waves called gamma oscillations. In healthy people, these bursts of neural activity tend to crop up in response to a stimulus — such as sights or sounds — and are thought to be important for focus and consciousness.
Individuals with autism and schizophrenia, however, seem to have high baseline levels of gamma waves, according to some studies3.
In the new study, Deisseroth showed that boosting excitatory activity in the prefrontal cortex, in addition to altering social behavior, also induces gamma bursts. “It was interesting to see that the same simple change can cause these two features that have both been linked to autism,” Deisseroth says. Whether the gamma waves contribute to social function or are a marker of it is unclear, however.
Optogenetics is not good for studying the whole brain at once, or changes that develop over long periods of time. Still, the approach is ideal for tweaking the activity of specific circuits while measuring behavior, notes George Augustine, an optogenetics expert and director of the Center for Functional Connectomics at the Korea Institute of Science and Technology in Seoul.
The new study gives a glimpse of the future of the field: using large libraries of opsins that allow researchers to tweak different kinds of cells simultaneously.
In an upcoming publication, Augustine says he and Deisseroth will debut four new mutant mouse lines that carry opsins in different kinds of brain cells. Collaborating with several other researchers, including Guoping Feng at the Massachusetts Institute of Technology, they are making about ten other mouse types. Augustine says several mutant lines have so far been shipped to more than 100 laboratories around the world.
“This is just the opening salvo in a whole new attack on psychiatric disorders,” Augustine says.
Recommended reading
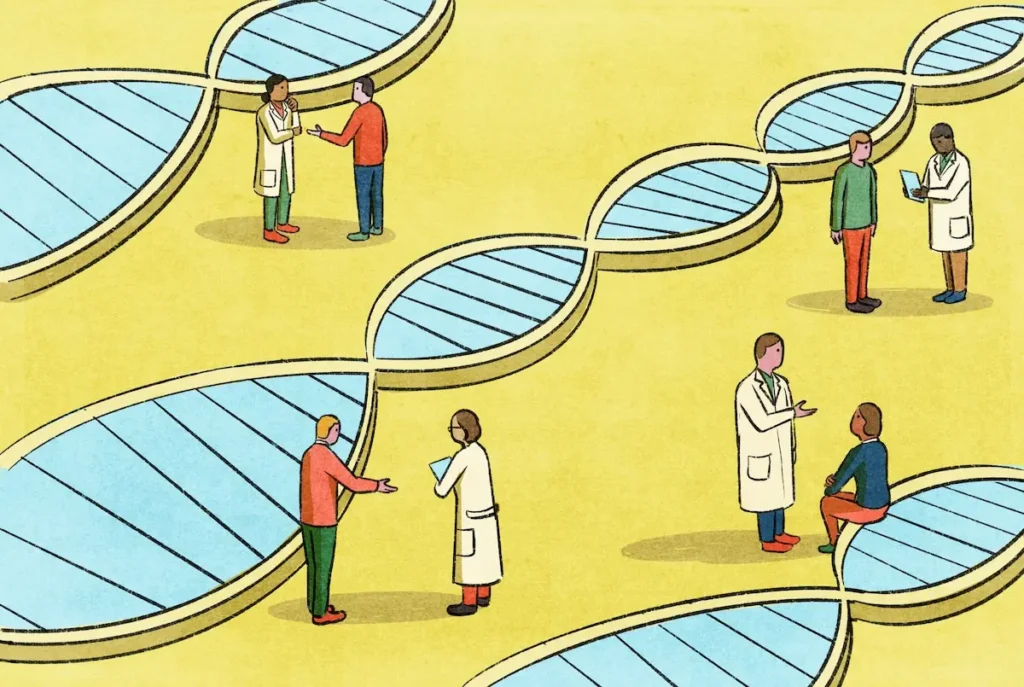
Building an autism research registry: Q&A with Tony Charman
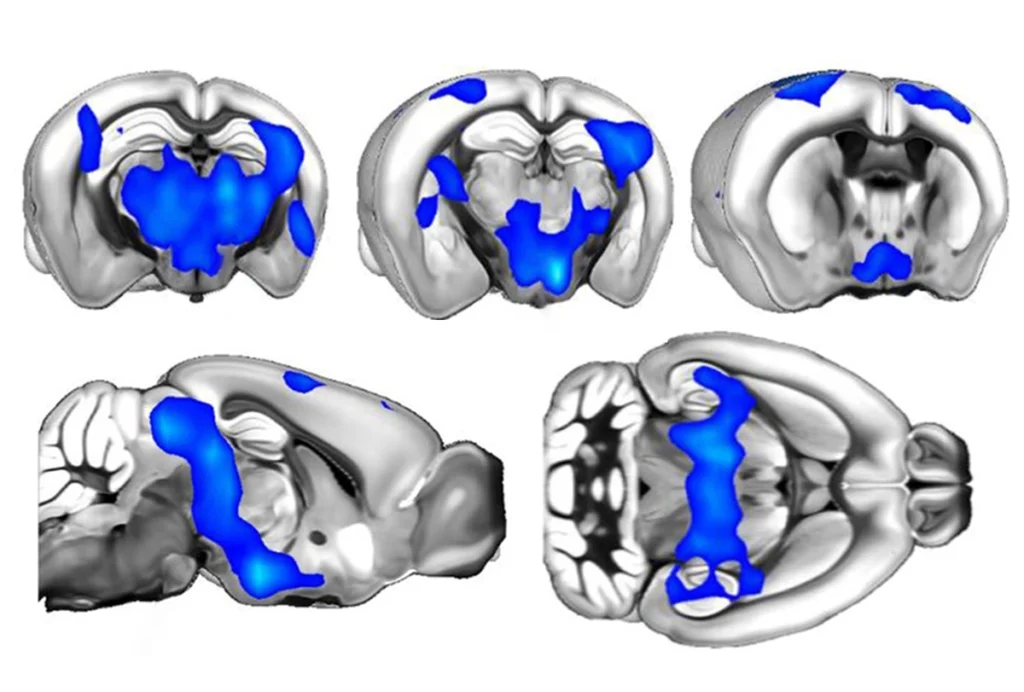
CNTNAP2 variants; trait trajectories; sensory reactivity
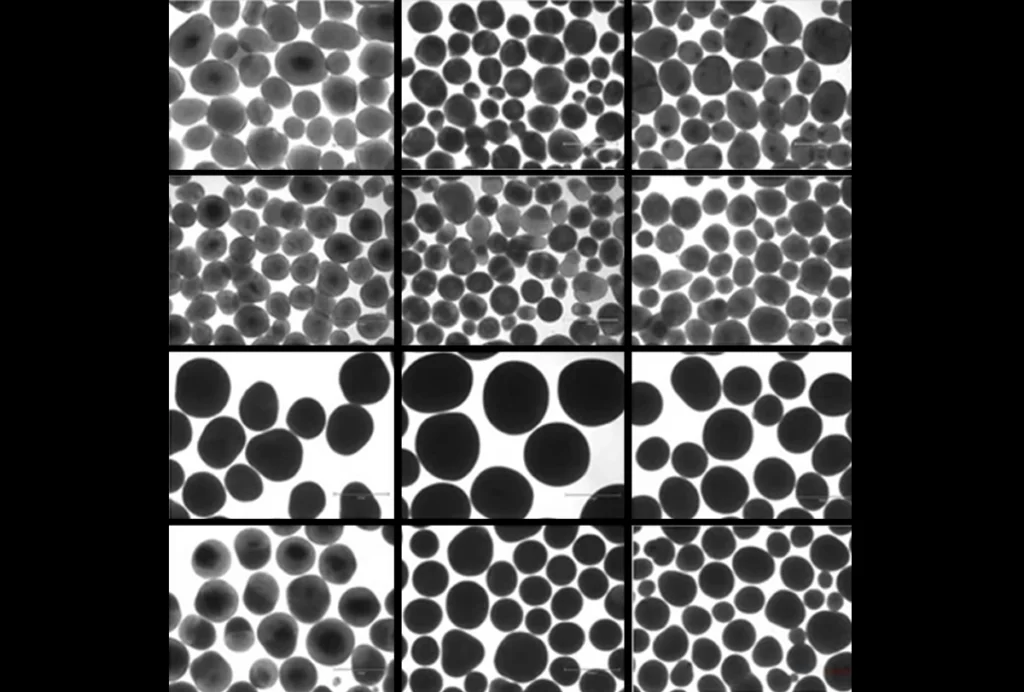
Brain organoid size matches intensity of social problems in autistic people
Explore more from The Transmitter
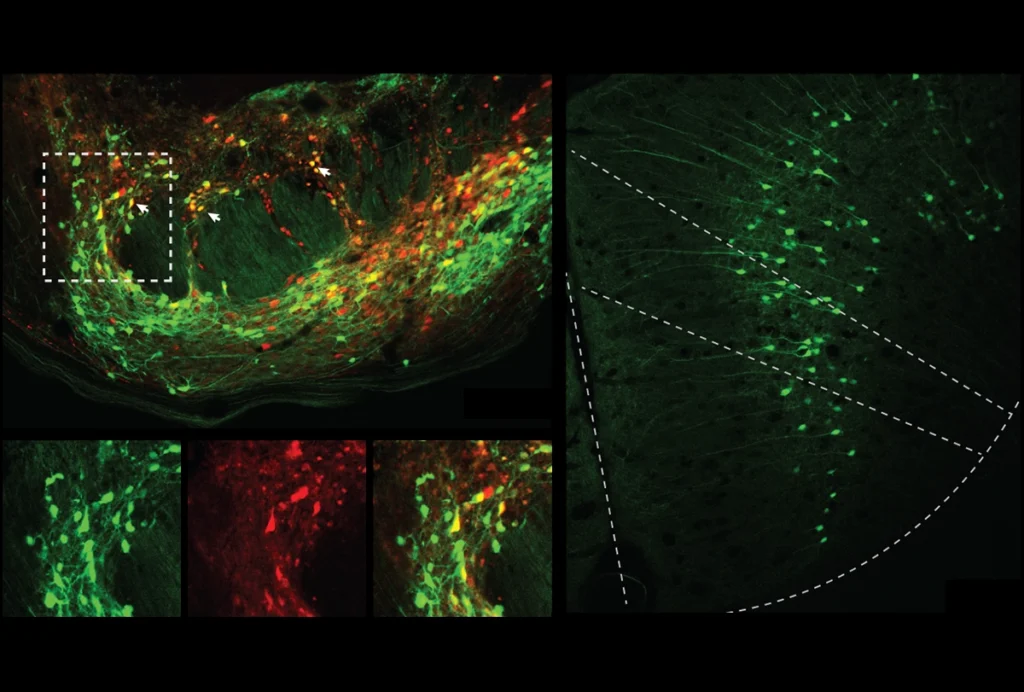
Cerebellar circuit may convert expected pain relief into real thing
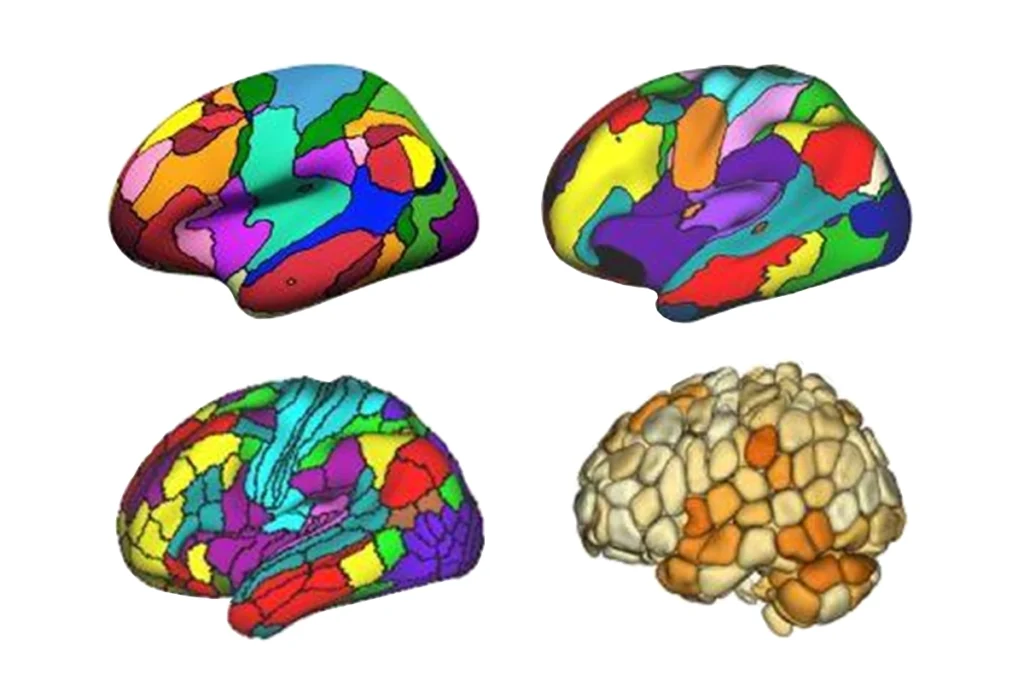