‘Optogenetics’ sheds light on role of different neurons
For decades, those who study brain cell activity have faced a fundamental trade off: either closely monitor the activity of a single cell or look at the circuit level to see how large groups of neurons communicate with each other.
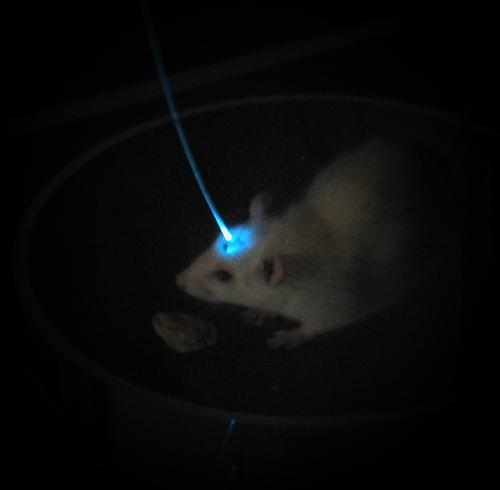
For decades, those who study brain cell activity have faced a fundamental trade off: either closely monitor the activity of a single cell or look at the circuit level to see how large groups of neurons communicate with each other.
A new technique, optogenetics, makes it possible to study both the micro and the macro levels of brain activity.
The technique allows scientists to stimulate activity in specific types of neurons with light, rather than with invasive metal electrodes.
Stanford University bioengineer Karl Deisseroth, who has used optogenetics in live mouse models since 2007, described the technique Saturday morning at the Society for Neuroscience conference in Washington, D.C.
Scientists first insert a gene that encodes a membrane protein ― belonging to a microbial class called ‘opsins’ ― into the brain tissue of mice or rats. Within a few days, the targeted brain cells take up the gene and begin expressing opsins.
Opsins are sensitive to light. For instance, if the protein channelrhodopsin-2 (ChR2) is taken up by mouse neurons, scientists can induce the cells to fire just by exposing them to a light source. The light is typically from a fiber optic cable that receives radiation from a laser, filters out electrical interference and heat, and delivers pure light through a surgical shunt into the targeted brain tissue.
The technology “is probably the biggest technical breakthrough in neuroscience this decade,” says Tony Zador, a researcher at Cold Spring Harbor Laboratory who also uses ChR2 to study neuronal connections in mouse models of autism.
The technique may be useful in observing the way neurons communicate in various mouse models of disease, including Parkinson’s disease, epilepsy and autism.
“My personal mission is to end up understanding how dynamic circuit systems are altered in neuropsychiatric disease,” Deisseroth says.
Orchestrating neurons:
In 1979, Francis Crick told Scientific American what he thought was one of the biggest challenges facing neuroscience: the ability to control only one type of neuron in the working brain, leaving the others more or less unaltered. That challenge remains today.
The brain is like an orchestra, Deisseroth says, and each sub-region a different type of musical instrument. “Basically we have no hope of understanding the brain until we can play the role of the conductor and drive different cell types,” he says.
Deisseroth’s team was the first to play conductor in a live animal brain. Last year, he and his colleagues published a flurry of papers on the optogenetics technique. The first, in September 2007, showed that when ChR2 is injected into specific neurons of the motor cortex in mice or rats, light stimulation can drive the animals’ whisker movements1.
The researchers were also able to induce mice to wake up from a sleep state by stimulating a specific type of neurons in the hypothalamus, the brain region that regulates sleep cycles2.
When ChR2 is injected into the right side of a mouse’s motor cortex, the brain region that controls movement on the left side, light stimulation forces the mice to move to veer left, running in counterclockwise circles3.
Deisseroth is now probing sociability in the neuroligin-4 mouse model of autism, as well as mouse models of Timothy syndrome ― a developmental disorder with some similarities to autism ― and Williams syndrome, which is characterized by extreme sociability.
In the experiments, a juvenile mutant mouse, after going through the optogenetic procedure, enters a rectangular chamber. On one side of the chamber is a passageway to a space that contains objects; on the other side is a passageway to a space that contains other mice. The tests aim to find out whether scientists can control which chamber the mouse chooses to explore just by stimulating the right type of neurons with light.
“The idea is that with this technique, we’re able to really tune sociability,” Deisseroth says. “Hopefully, we’ll get to a circuit-level understanding of that sociability drive.”
Long-range connections:
Zador is using the technique in mouse models of autism to study how neurons in different brain regions communicate with one another.
“Our hypothesis is that a major mechanism common to many autism genes is that they disrupt long-range connections,” Zador says. His team is injecting the ChR2 gene into one brain area, stimulating it with light, and then recording any activity produced in other brain areas.
Some researchers have also tried stimulating neurons in people to treat certain diseases.
For instance, in a technique called deep-brain stimulation (DBS), neurosurgeons insert a skinny metal electrode into select brain regions of people with Parkinsonian tremors, epilepsy, depression or obsessive-compulsive disorder. The technique has been shown to reduce many of the symptoms of these disorders. However, because the technique can’t target specific neuron types, there are also many unwanted side effects.
The optogenetic method might also be useful in treating eye disorders. In 2006, scientists stimulated the visual cortex in mice that lacked functional light receptors by inserting the ChR2 gene directly into their retinal cells. The technique may be useful in treating human retinal degeneration4.
Last year, Israeli researchers also proposed using the technique in neuroprosthetic devices for blind people, bypassing their non-working eyes to directly stimulate the deeper ganglion cells5.
Deisseroth is more cautious about human studies: because mouse and human immune systems are very different, humans could have strong immune reactions when exposed to the foreign opsin proteins, he notes. Scientists must also consider the ethical and philosophic issues of this form of human mind control, he adds.
“Nothing would completely prevent it from being translated into people, but we are not really pushing that part, just because we think there’s still so much to learn in animal models,” he says. “That knowledge alone will help us in understanding treatment.”
For a list of all news stories from the Society for Neuroscience annual meeting, click here.
References:
- Aravanis A.M. et al. J. Neural Eng. 4, S143-S156 (2007) PubMed
- Adamantidis A.R. et al. Nature 450, 420-424 (2007) PubMed
- Gradinaru V. et al. J. Neurosci. 27, 14231-14238 (2007) PubMed
- Bi A. et al. Neuron 50, 23-33 (2006) PubMed
- Farah N. et al. Conf. Proc. IEEE Eng. Med. Biol. Soc. 2007, 6368-6370 (2007) PubMed
Recommended reading
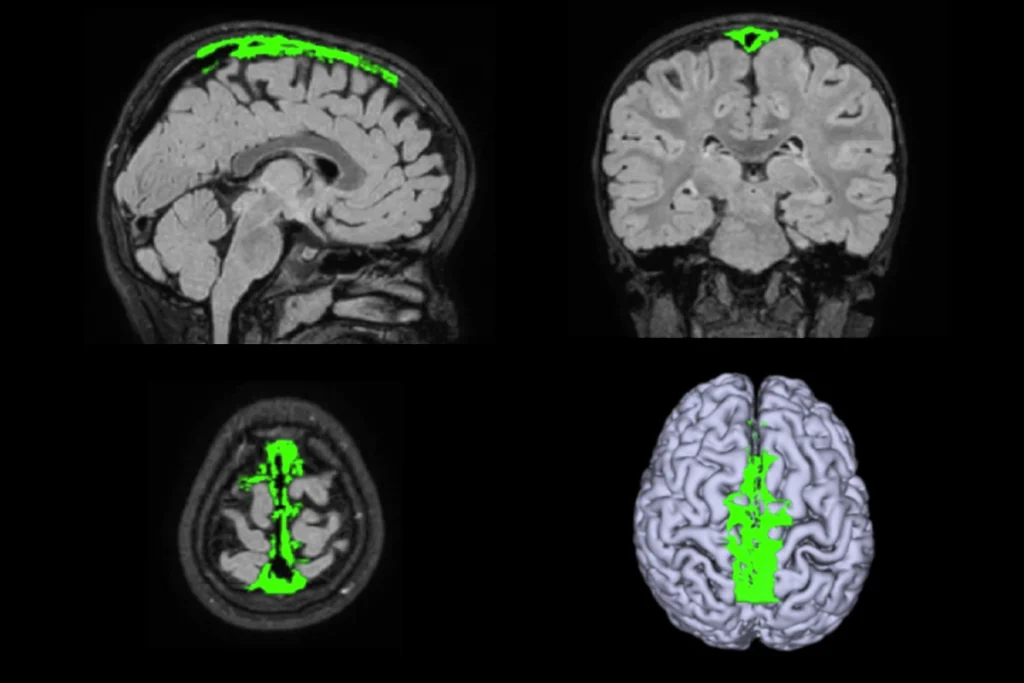
Okur-Chung neurodevelopmental syndrome; excess CSF; autistic girls
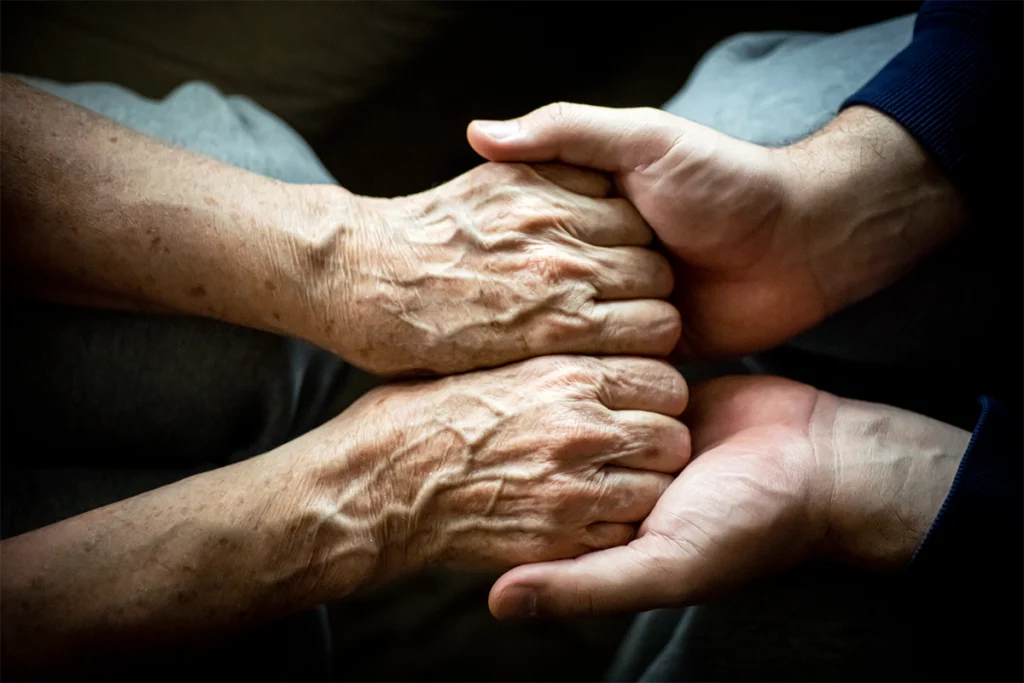
New catalog charts familial ties from autism to 90 other conditions
Explore more from The Transmitter
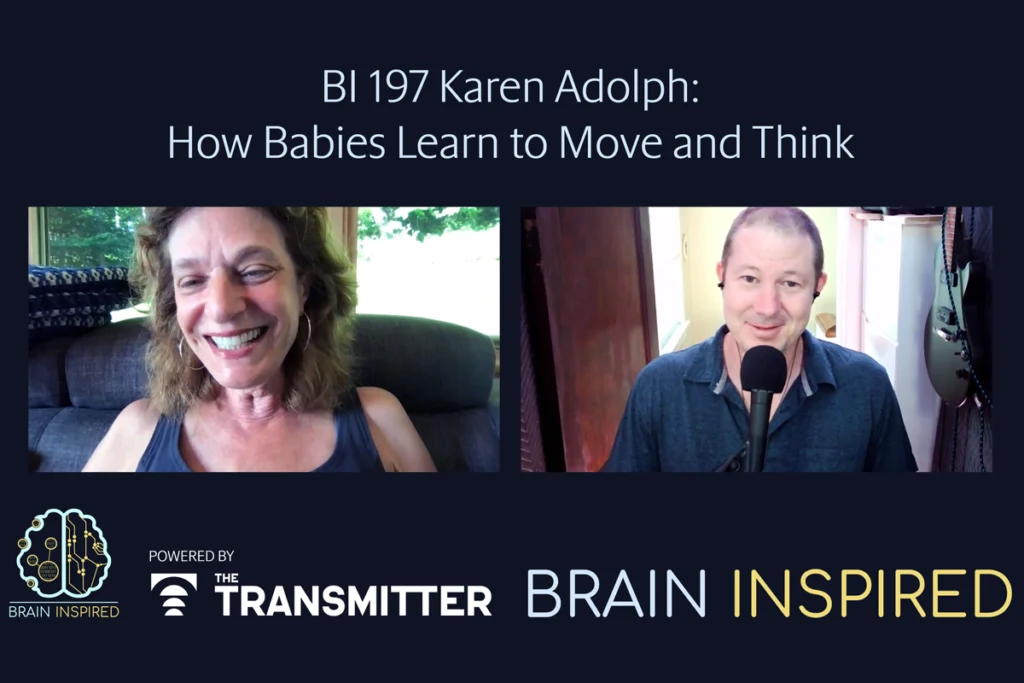
Karen Adolph explains how we develop our ability to move through the world
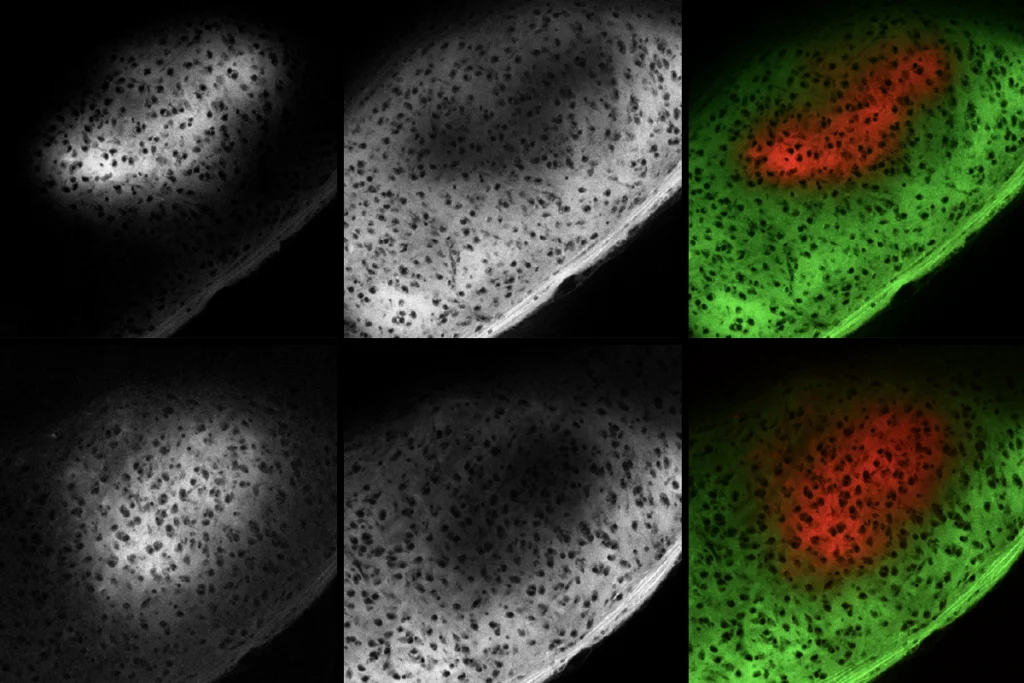
Microglia’s pruning function called into question
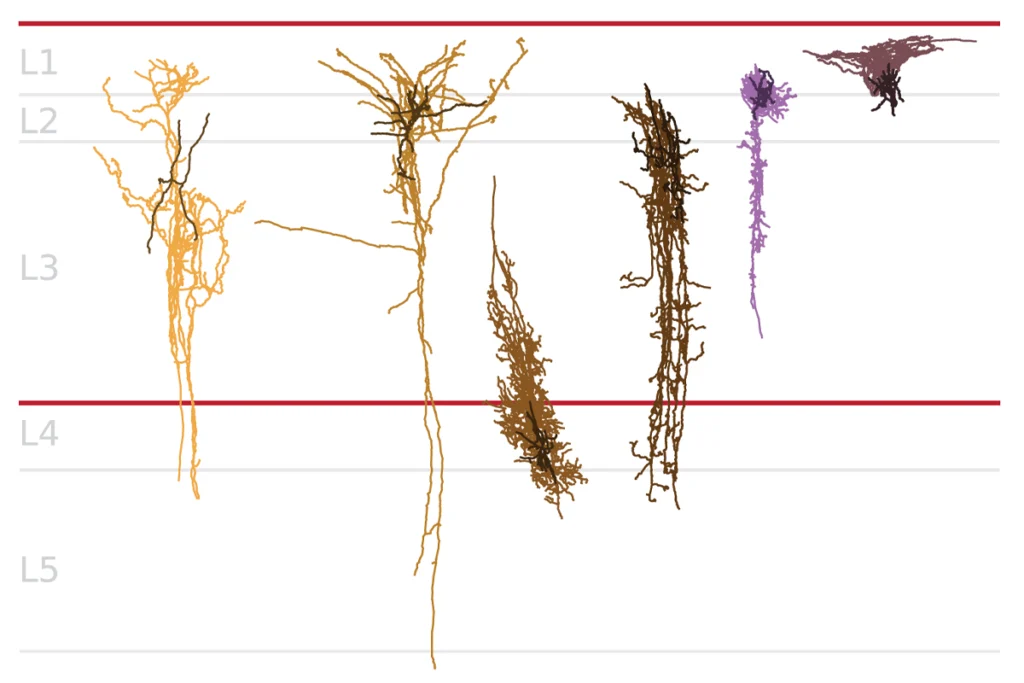