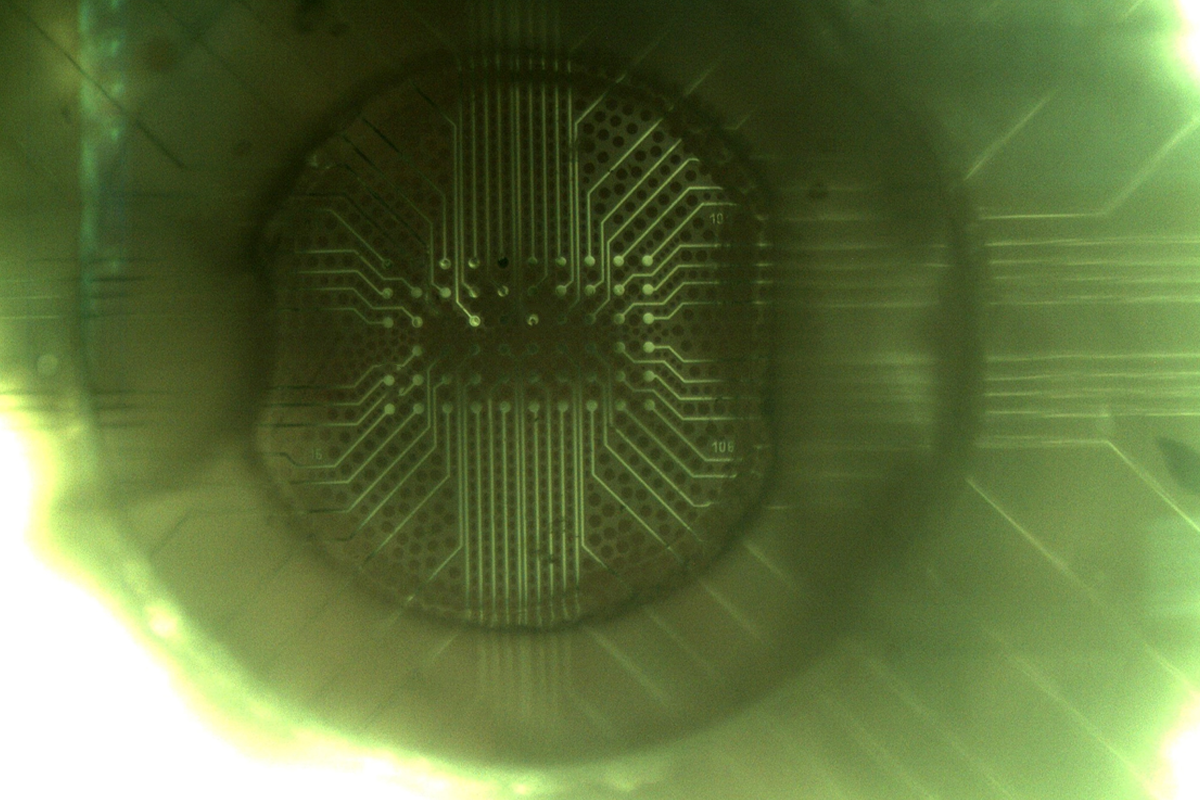
Making cancer nervous
Nerve cells in the brain and throughout the body can turbocharge tumor growth — a finding that not only expands conventional ideas about the nervous system but points to novel therapeutic targets for a range of malignancies.
Paola Vermeer remembers the moment in June 2019 clearly: She was standing with a cluster of colleagues around a fresh slice of a tumor removed just minutes before from a mouse model of head-and-neck cancer. It was now resting atop a microelectrode array, a device for recording electrical signals generated by living tissues. The device, though widely used in neuroscience, was an unfamiliar one for Vermeer, a cancer biologist at Sanford Research in Sioux Falls, South Dakota. But she needed it to test a hunch.
Several years earlier, Vermeer had become interested in the nerves that are often seen within cancerous tumors. Mounting evidence suggested that neuronal activity could promote tumor growth, but exactly how was still in question. “Very naively, I thought, ‘Well, nerves are electrically connecting cells,’” she says. “And so if they’re functional, there should be an electrical readout.”
Now, after a few training sessions from a Sanford colleague who uses microelectrode arrays to study mouse brains — a more typical application of the technique — and a handful of trial-and-error drills on her own, Vermeer had arrived at the moment of truth. The team gathered around the hulking microelectrode rig: a glass slide embedded with dozens of tiny electrodes and placed on the stage of a powerful microscope, surrounded by a wire cage to block other sources of electromagnetic radiation from ruining the experiment. Vermeer felt doubts creeping in.
“We put our first slice on the array, and in my head, I was like ‘Yeah, this isn’t going to work. There’s no way this is gonna work,’” Vermeer says.
The team gazed at a nearby computer screen showing a calendar-like grid of 60 boxes, representing each of the electrodes in the array. Fuzzy blue lines bisected the boxes horizontally, indicating the tissue’s weak baseline electrical activity. Then Vermeer pressed a button on the computer to stimulate an electrode on the edge of the array. Soon, wild spikes of cobalt blue began to propagate through the boxes on the screen, exposing strong electrical signals transiting through the tumor slice.
“When we saw activity, literally, my jaw dropped,” Vermeer says. “No way,” she thought. Here was evidence that nerves within malignant tissue are fully functional, akin to nerves in healthy tissues throughout the body.
Her next thought: “We need more tumors; we need to get more samples,” she says. For help with that, she turned to her collaborator and brother, Ronny Drapkin, a pathologist at the University of Pennsylvania in Philadelphia focused on gynecologic cancers, and director of an ovarian cancer biobank. Over the following months, he sent multiple shipments of live tumor tissue, bathed in transplant medium, overnight to South Dakota.
With more samples, the team demonstrated that nerves form functional circuits in both head-and-neck and ovarian tumors, and that tumors are more electrically active than normal tissues. Those results, described in a paper in Science Advances this past May, provide some of the strongest evidence yet that electrical signals drive tumor growth outside the central nervous system. The work also serves as an important extension of two independent reports in 2019 describing functional synapses within brain cancers called gliomas.
Together these findings bolster the emerging field of cancer neuroscience. Though barely more than two decades old, cancer neuroscience is already a sprawling field, dealing broadly with the cross-talk between malignant tumors and neurons in both the central and peripheral nervous systems. It encompasses topics ranging from sleep disruptions in people with cancer to the cognitive effects of chemotherapy.
“Most of the evidence, and really the center of the field, is around the idea that neurons and nerves” — via a variety of mechanisms that researchers are still teasing apart — “seem to regulate almost everything about cancer, from tumor initiation in many cases to tumor growth, tumor invasion and metastasis, probably resistance to therapy, and evolution of the disease,” says Michelle Monje, a neuroscientist and neuro-oncologist at Stanford University in California, who headed one of the teams that found synapses in gliomas.
The field sits at the intersection of two broad themes in contemporary biomedical science: the rising awareness that the nervous system guides the development of organs and tissues throughout the body, and the understanding that tumors are not just undifferentiated lumps of cells, but neo-organs in their own right.
In their interactions with neurons that drive tumor growth and progression, cancer cells are “not inventing anything new. They’re just using these mechanisms of development and plasticity” that are fundamental to normal body processes, Monje says. By studying the interactions between the nervous system and cancer, Monje, Vermeer and a growing cadre of researchers aim to learn how to treat cancer more successfully, as well as gain basic insights into the normal development and function of the nervous system.
P
athologists first spotted nerve cells lurking in tumors in the early 1900s. But their observations did not capture the attention of cancer biologists for another 100 years or so, Drapkin says. The cancer biologists tended to regard the cells as clinical curiosities, mere bystanders rather than active participants in malignancy.Then, in 1998, a professional setback for one pathologist turned into a lucky break for the field. Gustavo Ayala had just moved from the recently defunct Allegheny University of the Health Sciences in Philadelphia, Pennsylvania to Baylor College of Medicine in Houston, Texas, and he needed a project to keep him busy while he waited for his state medical license to come through. He decided to look into perineural invasion, or the tendency for cancer cells to cluster around local nerve fibers.
“Of course, I knew about perineural invasion as a pathologist. It’s very common,” says Ayala, who is now at the University of Texas at Houston. He also knew that tumors that exhibit perineural invasion tend to be more deadly; the cancer cells use the nerves as highways to exit a primary tumor site and metastasize. “What stood out was that it was clinically very important. But nobody knew why. And nobody knew how,” he says. “So I decided to make that my research.”
Ayala developed a way to grow prostate cancer cells in laboratory dishes together with mouse dorsal root ganglia — clusters of neurons that sit just outside the spinal cord and connect the peripheral and central nervous systems. “It was pretty amazing. I mean, things happened immediately,” Ayala says, describing the method. Within 24 hours of landing in the dish, the neurons sent out long, thin filaments called neurites in the direction of the cancer cells.
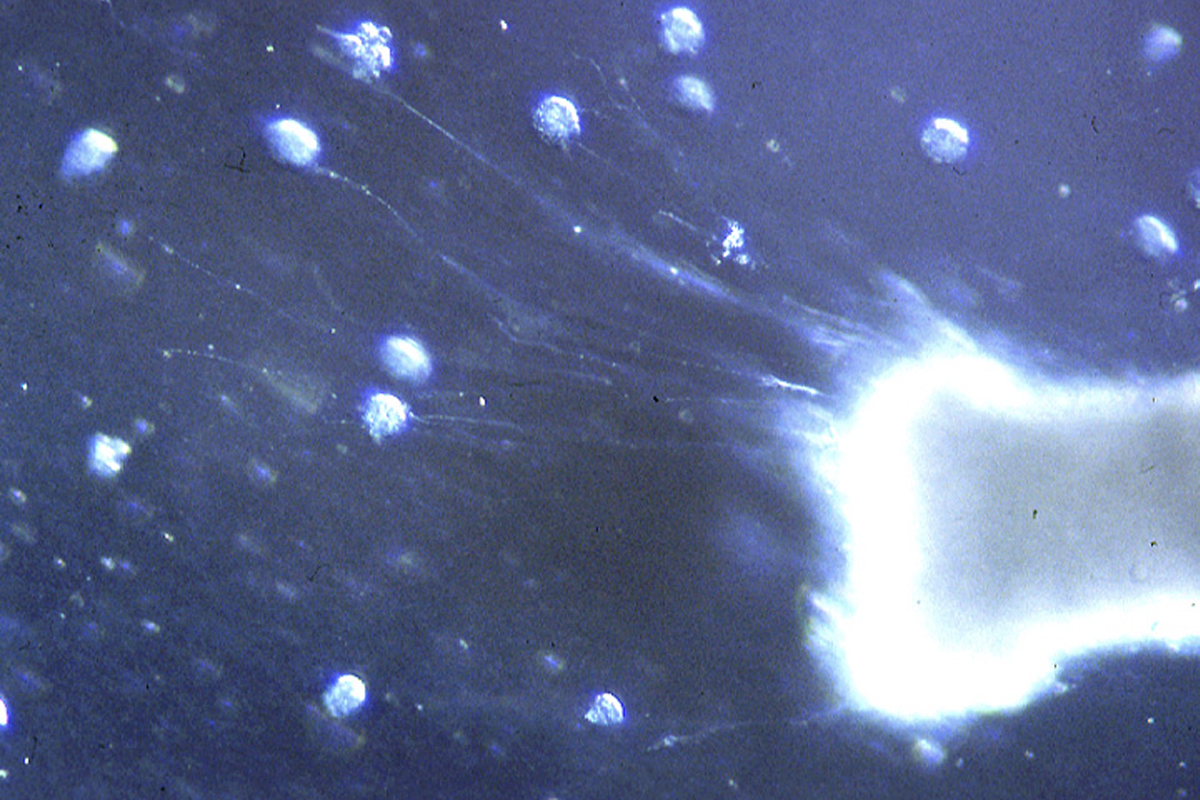
Ayala remembers showing his results to his department chair on Kodachrome slides, enlarging the images of the cells to larger than life size on the wall above the chair’s office door. In one of these early images, scattered colonies of prostate cancer cells appear as bright planetary dots against a background of deep blue. The mouse ganglion in Ayala’s dish glows on the right side of the frame, and luminous tendrils of neurites extend from it toward the cancer-cell colonies like solar flares. “He looked at me,” Ayala recalls, “and he said, ‘There’s biology there. Follow this.’”
Ayala did, and in 2001, he published his findings on what he describes as a “symbiosis” between neurons and cancer cells. “The neurites have directional growth towards the cancer cells. And the cancer cells grow more in the presence of the neurites,” he says. “The neurons grow more in the presence of the cancer cells, and the cancer cells eventually track along the neurites and wrap around the big nerve and the dorsal root ganglion.”
Working on neuron-cancer interactions “was lonely at the beginning,” Ayala says. But he persevered, first identifying how perineural invasion lends a growth and survival advantage to prostate cancer cells and later observing a greater density of nerves in cancerous prostates, among other studies. As cancer biologists increasingly began to view tumors as neo-organs, they expanded their study of the tumor microenvironment from predominantly blood vessels and immune cells to nerve cells. And gradually, other clinical and epidemiological links between cancer and nervous system function started to accumulate.
In the 2000s, for example, some studies linked a lowered risk of cancer progression or death with taking beta blockers — drugs that dampen signals from a certain class of nerve fibers and are widely used to treat high blood pressure and heart disease. Then, in the mid-2010s, a series of mouse studies showed that cutting or blocking nerves innervating prostate and gastric tumors could stop cancer in its tracks.
Those results began to draw more scientists to the field.
S
oon, the rapid proliferation of studies made the picture of neuron-cancer interactions more complex — not only in terms of what nerve cells in tumors actually do, but also how they get there.Scientists now believe that nerve cells are a feature of solid tumors throughout the body and brain. But in contrast to what early studies suggested, those nerves don’t universally stimulate cancer. Many tumors host multiple types of nerve fibers, including some that can spur and some that can dampen growth. Particular classes of nerve fibers seem to have different effects, too, depending on a tumor’s location.
“In every organ, the interaction is slightly different, and the role of different types of nerves slightly different,” says Timothy Wang, a gastroenterologist and gastrointestinal cancer researcher at Columbia University, who co-led the study of denervation to block gastric cancer.
For instance, sympathetic nerves — part of the autonomic nervous system responsible for the “fight or flight” response — stimulate the growth of breast and pancreatic tumors. But parasympathetic nerves — responsible for the body’s “rest and digest” functions — suppress the growth of these two cancers. Gastric cancer shows the opposite pattern: Sympathetic activity dampens its growth, and parasympathetic activity drives it. And both sympathetic and parasympathetic activity promote the growth of prostate cancer.
Sensory nerves, which relay information from the periphery to the central nervous system, also promote the growth of breast, cervical, head-and-neck, ovarian, pancreatic and skin cancers, research shows. “The sensory nerves are particularly interesting because they have many different neurotransmitters, and every organ has a little different mix of the different types of sensory nerves [it contains],” Wang says.
Studies of sensory nerves suggest the influence between the nervous system and tumors may run both ways. At least some sensory fibers within tumors track back to dorsal root ganglia, according to experiments by Drapkin and Vermeer in which they used a genetically engineered virus to map that path in mouse ovarian tumors. And pain fibers in a mouse model of head-and-neck cancer appear to link tumors to preexisting circuits in the brain, altering the activity of the brain circuit and even the behavior of the mice, Vermeer and her colleagues reported in a preprint posted on bioRxiv last month.
“I think that’s an unbelievably exciting emerging concept — that there could be not just innervation of tumors, but there may be connections between the CNS and the periphery,” Drapkin says. Such links could help explain changes in mood and appetite, and other quality-of-life issues that are common in people with cancer, he adds.
Often, the mix of nerves found in tumors reflects the local nerve population, which in turn reflects the developmental biology of those tissues. “I think rather than telling you about how cancers are different, in a way it tells you about how the initial progenitors in those organs are probably regulated to start with,” Wang says. A tumor “simply takes advantage of that particular pattern of neural innovation and then uses it” for its own ends. But some tumor nerves have other sources. For instance, although sensory nerves are prevalent in ovarian tumors, the fallopian tubes and ovaries contain mostly sympathetic fibers.
Mounting evidence suggests that neurons sometimes arise from a tumor itself: Tumors contain cancer stem cells, which have the capacity to differentiate in various directions and sometimes acquire neuronal markers, studies show. Some evidence hints that fully differentiated cancer cells can be reprogrammed into neurons, or acquire neuron-like traits to liberate late-phase tumors from any dependence on outside neurons.
And there is still another possibility, according to a line of inquiry led by Claire Magnon, research director at the French National Institute of Health and Medical Research (INSERM) in Paris: Some nerves in tumors may derive from neural progenitor cells that migrate from the brain.
When Magnon set up her own lab in 2015, she decided to pursue a 2008 finding from Ayala showing increased neurons in the prostate ganglia of people with cancer. One figure in that paper, a simple histogram of neuron numbers, was particularly striking, she says. “I kept that in my mind for months.”
In prostate tumor samples from both people and mice, Magnon soon found cells that express doublecortin, which can be a marker of immature neurons. Molecularly, the cells were most similar to neural progenitors in a brain region called the subventricular zone (SVZ). Surprisingly, the mice bearing prostate tumors had fewer of these doublecortin progenitors in the SVZ than tumor-free mice did. And the number of progenitors in the SVZ declined over time as the animals’ prostate tumors developed.
That was tantalizing evidence, but still circumstantial. Magnon wanted to be sure, so she kept designing more experiments. Then one evening, as she peered at slides of mouse tumor samples under the microscope, she spotted cells glowing with the lurid red of tdTomato, an exceptionally bright red fluorescent protein. Weeks before, she had injected a virus containing the tdTomato gene into the mouse’s SVZ. Now, through her eyepiece, she could see the cells from the brain that had taken up the gene, slipped into the bloodstream and relocated to the tumor.
“It was the final experiment for me. And I took a picture, and I sent it to one colleague,” Magnon says. “I was excited.” The picture became part of a figure in a paper that appeared in Nature in 2019. The findings suggested an unsettling possibility, Magnon and her colleagues wrote in that study: By drawing neural progenitors out of the brain, tumors may, in effect, steal the brain’s resilience, perhaps contributing to the cognitive impairments seen in some cancer survivors.
P
robing neuron-brain-cancer interactions, though “very, very obvious,” was stymied at first by technological barriers, says Humsa Venkatesh, a neuroscientist at Harvard University. “We didn’t really have a great way of modulating neuronal activity in the brain.” But widespread diffusion of calcium imaging, optogenetics and chemogenetics led to a surge of studies beginning in the early 2010s.In late 2015, Monje traveled to Germany to give a talk about some of those findings at a symposium organized by Frank Winkler, a neuroscientist at the University of Heidelberg in Germany. The two had never met, but sitting in Winkler’s office before Monje’s talk, they fell into easy conversation, chatting about the practice of neurology, family (Monje was about five months pregnant with her fourth child) and the research going on in their respective labs.
“I said, ‘Michelle, so we just discovered something really crazy,’” Winkler recalls. “And then she said, ‘Well, we discovered something really crazy.’” And then I think it took us 10 minutes or so to figure out that we’ve discovered the same thing.”
Both labs had been building a story of how tumor cells link with each other and with the rest of the brain to drive brain cancer. Researchers in Monje’s lab had accelerated the growth of human glioma cells implanted in mouse brains by stimulating neighboring neurons with light, and traced the effect in part to a molecule called NLGN3. “It was a really dramatic effect,” says Venkatesh, who worked on the studies as a graduate student in Monje’s lab. “In the absence of this one single protein alone, the tumors just did not grow.”
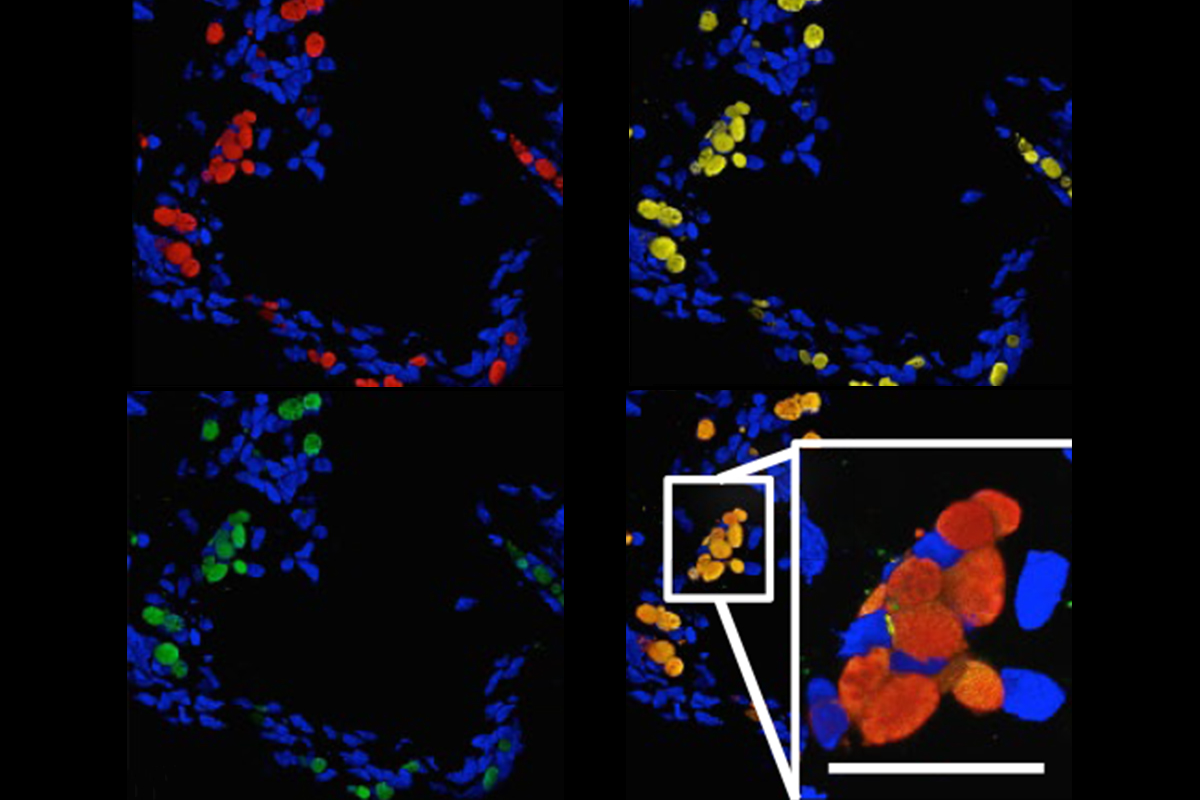
Winkler’s lab, meanwhile, had been investigating long, neurite-like extensions, dubbed “microtubes,” on brain cancer cells. These microtubes, they found, enabled tumor cells to form a network, linked by connections called gap junctions. The networked tumor cells, they also discovered, are resistant to radiation, surgery and chemotherapy.
Now the latest results from both labs had suggested something even more profound: Brain tumors and neurons form functional synapses with each other. “We were both kind of hinting at it,” Monje remembers of that conversation in Winkler’s office. “And there was a moment where I looked at him and said, ‘You know, I think there are synapses. Do you also think there are synapses?’ And he said, ‘I do.’ And I said, ‘Oh my gosh!’”
It could have been a tense, competitive moment. Instead, the two opted to view it as a scientific bonus: Each team’s work had already been independently validated. “If something really crazy is discovered on both sides of the Atlantic Ocean, it’s probably not completely untrue or completely made up,” Winkler says.
The two stayed in touch, shared data and wound up reporting their results in a pair of papers published simultaneously in Nature in 2019.
A brain tumor “is not just responding to signals around it. It’s actually integrating into the neural circuits of the brain, and that is a very different way of thinking about a cancer,” Monje says. “The activity of someone’s own brain may influence growth and progression of the cancer that will ultimately take their life.”
Brain cancer actually remodels brain circuits, several research groups have shown. In glioblastomas, for instance, tumor cells increase the excitability of the surrounding neurons by secreting a molecule that promotes the formation of synapses, according to work from Benjamin Deneen, a neuroscientist at Baylor College of Medicine, and his colleagues. “You have this vicious, self-propagating cycle,” Deneen says. “The tumor is sitting here in a bed of neurons. And the tumor is basically just dumping out factors that are making the neurons become more and more and more active.” This cycle may also help explain why so many people with brain tumors develop seizures, he adds.
Brain cancer cells secrete another molecule, according to work published this year by Monje, Venkatesh and other researchers, that not only promotes synapse formation on neurons connected to a tumor but also increases brain-tumor functional connectivity, or the degree to which they tend to activate in concert. The greater the functional connectivity, the more deadly the cancer, they reported. What’s more, gliomas hijack synaptic plasticity — a mechanism key to learning and memory — to become more strongly connected with the healthy brain, a separate group of researchers, also including Monje and Venkatesh, reported earlier this month.
Even more insidiously, brain tumors themselves appear to be able to provide the increased excitability they need to grow, Winkler and his colleagues reported in January. A small number of tumor cells, which the team dubbed “pacemaker” cells, generate spontaneous pulses of electrical activity and can drive tumor progression all on their own.
Some of these mechanisms may also contribute to devastating brain metastases of peripheral cancers. Breast cancer cells introduced to the brain form indirect connections with neurons there, enabling neuronal activity to nourish the growth of the metastasis, according to one 2019 mouse study. And in a preprint posted on bioRxiv in January, Venkatesh and her collaborators detailed how neuronal activity helps small-cell lung cancer cells grow in the brain. “The neurons themselves and neuronal activity itself can actually modify the [lung cancer] cells,” Venkatesh says — upregulating certain receptors to better enable the tumor cells to receive neuronal cues.
A
s researchers begin to decode the cross-talk between nerves and cancer, the molecules involved are offering new targets for treatments. Some target tumor nerves directly, but many others aim to counteract the nerve-stimulating effects of chemotherapy and radiation, or enhance the effectiveness of treatments targeting other elements of the tumor microenvironment, such as blood vessels and immune cells.And though some of these therapies are new — one experimental drug currently in a phase 1 clinical trial inhibits a molecule responsible for the release of NLGN3, the molecule that Monje and Venkatesh identified as being important in pediatric glioma, for example — existing drugs also offer a vast array of possibilities.
“There are more than 100 drugs that are approved in neurology, psychiatry [and] internal medicine that target neural signaling pathways,” says Winkler, who is involved in efforts to test two approved medications, meclofenamate and perampanel, in people with glioblastoma. Meclofenamate, a nonsteroidal anti-inflammatory drug, also inhibits gap junctions, the features he has found connect microtubes on tumor cells. Perampanel, an anti-seizure medication, has the potential to inhibit tumor growth by dampening hyperexcitability, according to the 2019 study of brain cancer synapses from Winkler’s lab.
The strategy of repurposing existing drugs is a pragmatic one, says Erica Sloan, a cancer researcher at Monash University in Melbourne, Australia. “Our recent research has been focused on how we can combine beta blockers with existing [cancer]treatments, because that’s the fastest way to have patients benefit,” she says.
A short course of beta blockers around the time of breast cancer surgery renders tumor cells less invasive and could help prevent metastasis, according to findings from a phase 2 clinical trial Sloan’s team published in 2020. “We also found that there were changes in the immune cells that got recruited into tumors,” she says. “We got many more of the good immune cells coming into tumors.” Beta blockers can reduce metastasis after anthracycline chemotherapy in a mouse model of triple-negative breast cancer as well, according to a study Sloan and her collaborators published earlier this year.
So far, most trials of potential cancer therapies targeting neural mechanisms are in their early phases, but already some animal studies suggest their power might ultimately exceed scientists’ expectations. Monje vividly remembers a conversation that helped fuel her own excitement in 2016. She had walked three-quarters of the way down a long hallway toward her lab, she says, when she received a call from David Gutmann, a physician and neuroscientist at Washington University in St. Louis, Missouri, who specializes in an inherited condition called neurofibromatosis type 1 (NF1).
Children with NF1, which arises from a mutation in the NF1 tumor suppressor gene, are prone to developing benign tumors called neurofibromas around peripheral nerves, and about 15 percent develop cancers called optic gliomas around the optic nerve, which carries visual information from the eye to the brain.
Monje and Gutmann were collaborating on a series of studies using an NF1 mouse model developed in Gutmann’s lab. The mice were known to develop optic gliomas beginning around 9 weeks of age. Researchers in Monje’s lab were investigating whether optogenetic stimulation of the optic nerve would accelerate the growth of those tumors. The opposite experiment was taking place in Gutmann’s lab: rearing a cohort of mice in the dark to try to dampen the tumor growth.
“That first cohort, we dark-reared the mice in David’s lab, and he called me, and he said, ‘There are no tumors,’” Monje remembers Gutmann telling her over the phone.
“What? What do you mean there are no tumors? Are you sure it’s the right mice?” she remembers responding. “And he’s like, ‘Yes. I’ve never seen this before. There are no tumors.’ And I said, ‘Well, that’s crazy. Let’s do it again.’ And so we did it here in my lab. We did it again in his lab. There were no tumors.” Gutmann was just as surprised, he says.
They worked to better understand the effect, reporting their findings in a paper published in Nature in 2021. The tumors’ chance to grow, they found, appears to be limited to a critical period in development, because mice that are subsequently returned to normal light-dark cycles do not later develop tumors. What’s more, if mice that have developed tumors at about 9 weeks old are placed in the dark between 12 and 16 weeks old, the growth of the optic nerve tumors slows.
The results in the mice do not translate directly into treatment for people with NF1, but they have provided some solid leads. NF1 neurons in mouse models, for example, are hyperexcitable — which drives the formation of both peripheral neurofibromas and central nervous system tumors, researchers from the two labs later found. They traced the hyperexcitability to a particular ion channel in the cell membrane. “When we found that it worked through that ion channel, we asked whether there were anti-epileptic drugs that would reverse that activity,” Gutmann says.
Gutmann aims to conduct a clinical trial of one of those drugs, lamotrigine, in children with NF1. Those results may take years still — but Monje thinks back to that early call from Gutmann that told her they were on the right path. “You know it’s a big memory when you know where you were when you got that call,” she says. “It was one of those moments that gave me, as a scientist, the sense of ‘OK, this is important. We have to understand this.’”
Explore more from The Transmitter
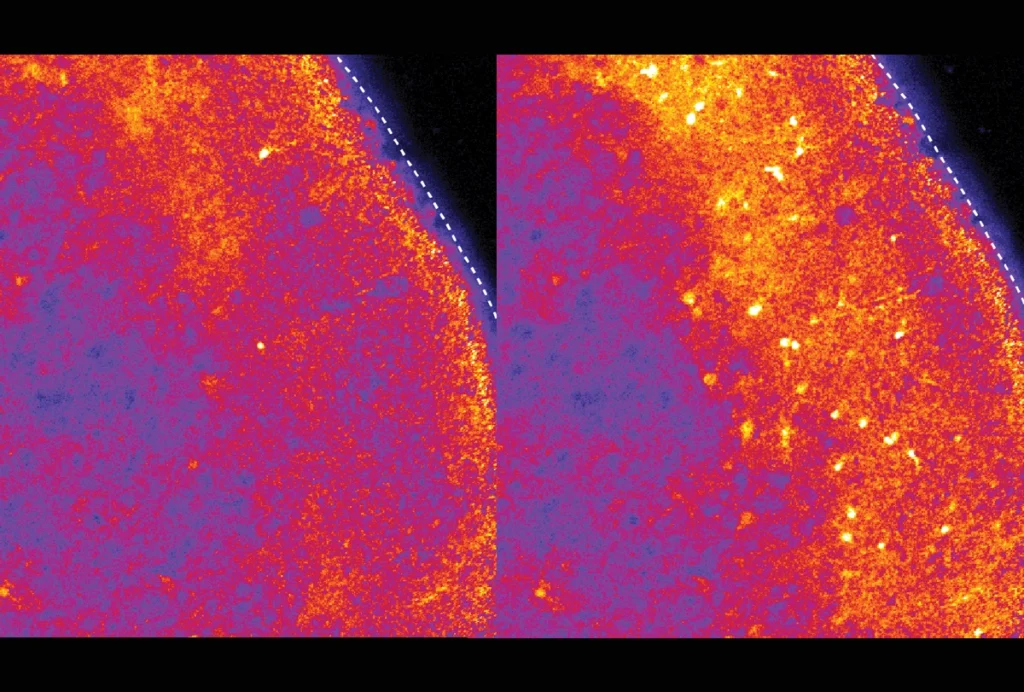
RNA drug corrects calcium signaling in chimeric model of Timothy syndrome
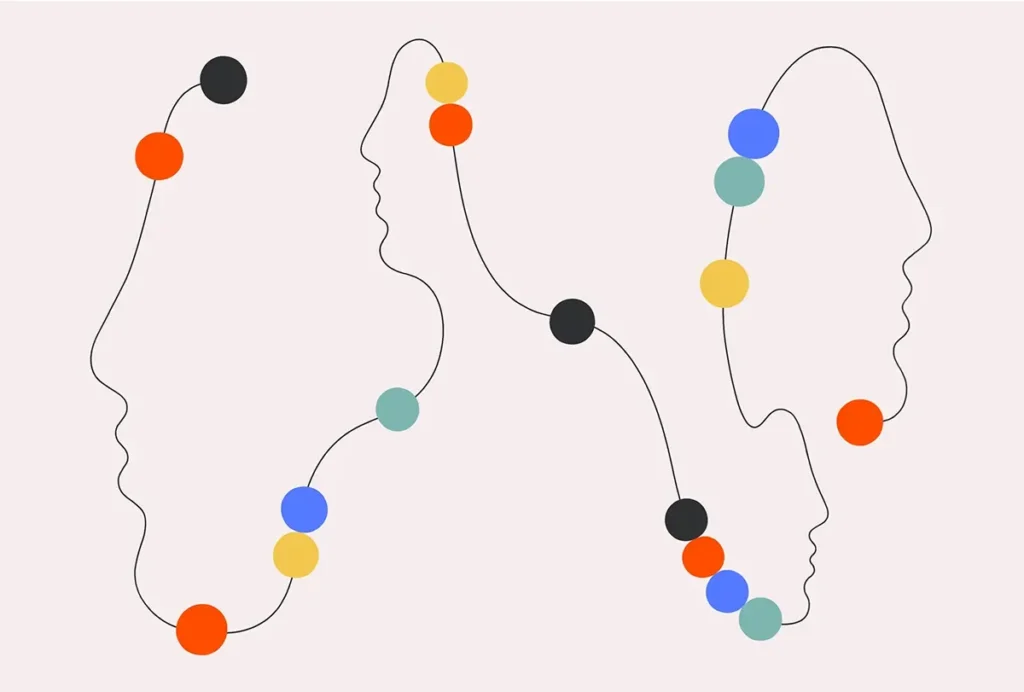