Future of autism genetics should learn from its past
To optimize the search for autism genes, researchers should collect large numbers of sequences — but the sequences need to be of the right kind, says Michael Ronemus.
Last month, my colleagues and I published two large studies that sequenced the genes or exomes of thousands of families with a history of autism1, 2. These studies identified several dozen ‘high-confidence’ autism genes that show spontaneous, harmful mutations in multiple affected (and unrelated) individuals.
Picking the ripest of these low-hanging fruits — through ‘brute-force’ genomics and discovery of spontaneous, or de novo, mutations — has brought us much closer to understanding the genetics of autism than we were just five years ago. But to optimize what we can learn, our studies must be informed by what we have already discovered. We certainly need many more sequences from more families with autism. But these sequences need to be of the right kind.
When large-scale genomic analysis began on cohorts of people with autism, we hoped — and expected — to discover many, if not most, of the underlying genes. We have undoubtedly found many, and perhaps even most, using the broadest criteria for an autism gene.
We can now put forth a statistically sound estimate of the overall genetic contribution of de novo mutations of different categories to autism. (These categories include loss-of-function mutations — which prevent full protein production — and missense variants, which have a less clear effect on protein function3.) There is little doubt that this approach remains the most powerful weapon in today’s arsenal —not just for autism spectrum disorders, but other neuropsychiatric and sporadic genetic disorders as well.
But we still cannot pinpoint the causal mutations for many cases of autism because the genomic background noise remains high: More than half of even the most damaging single hits to a protein are present in an individual by chance and are not linked to autism. Given this, it is not always clear what to tell clinicians and genetic counselors who are on the front line and wish to make use of these data.
So what is the future of family-based genomic studies of autism, with detection of de novo mutations as the central focus?
Where to look:
The good news is that we have truly begun to narrow the search. Put simply, we have a much better idea of where to look than we used to. The cost of sequencing continues to drop, and sequencing the entire genome is rapidly becoming a financially viable option.
These data will enrich the existing dataset by uncovering genomic regions that were not accessible using previous methods4, 5. Dropping costs will also allow us to sequence large numbers of gene candidates in large populations, as was done previously for smaller gene sets6.
“We still cannot pinpoint the causal mutations for many cases of autism because the genomic background noise remains high.”
However, in some ways our current approach may be reaching its limit. For example, the types of mutations highlighted in our two studies are more rare in males with autism who have intelligence quotients (IQs) above 90, and the genes affected largely do not overlap with the genes mutated in females or in males with autism who have IQs of 90 or below.
This suggests that another, relatively unexplored type of risk — such as common variants — perhaps with greater environmental contribution, may lead to autism in this group.
It’s also possible that we have already found the most important genetic contributors to autism. Simply extending the current discovery paradigm along existing lines might yield diminishing returns, despite the many candidate genes already identified.
There is legitimate reason to question whether groups containing a large proportion of high-functioning males will be informative, at least in the near term. Genetic efforts may be most effective if directed toward females and lower-IQ males with autism, who are the most likely to carry harmful mutations.
We also need to know where not to look. Almost all families in one well-characterized group, called the Simons Simplex Collection, include at least one unaffected sibling. (The collection is funded by the Simons Foundation, SFARI.org’s parent organization.)
This allowed us to do statistical analyses to calculate the overall likelihood of mutations being found in an individual by chance. It also allowed us exclude genes that are mutated in unaffected siblings. Without these data, the conclusions we reached would have been far murkier.
We can refine our analysis by applying statistical models to integrate information in inherited variation and studies that compare data from cases and controls with the existing de novo data. Data from people with other disorders are also an essential piece of the puzzle.
It is important to remember that these models need to account for the unique features of autism spectrum disorders. This includes well-established characteristics such as the high male-to-female gender ratio and the implication that females serve as carriers due to a protective effect.
It also includes more recent observations: for example, the lack of overlap in gene targets between males of high and low IQs and the finding that females have more deleterious mutations in genes expressed during embryonic development than would be expected by chance.
As a final note, the new studies also highlight the contribution of de novo ‘missense’ mutations, which have less clear effects on protein function. This relationship between strongly inactivating variants (harmful) and moderately inactivating variants (missense) needs to be understood at the functional level.
In many respects, the real fun is just beginning.
Michael Ronemus is research assistant professor at Cold Spring Harbor Laboratory in New York.
References:
1: Iossifov I. et al. Nature 515, 216-221 (2014) PubMed
2: Poultney, C.S. et al. Nature 515, 209-215, (2014) PubMed
3: Ronemus M. et al. Nat. Rev. Genet. 15,133-141 (2014) PubMed
4: Levy D. et al. Neuron 70, 886-897 (2011) PubMed
5: Sanders S.J. et al. Neuron 70, 863-885, (2011) PubMed
6: O’Roak B.J. et al. Science 338, 1619-1622 (2012) PubMed
Recommended reading
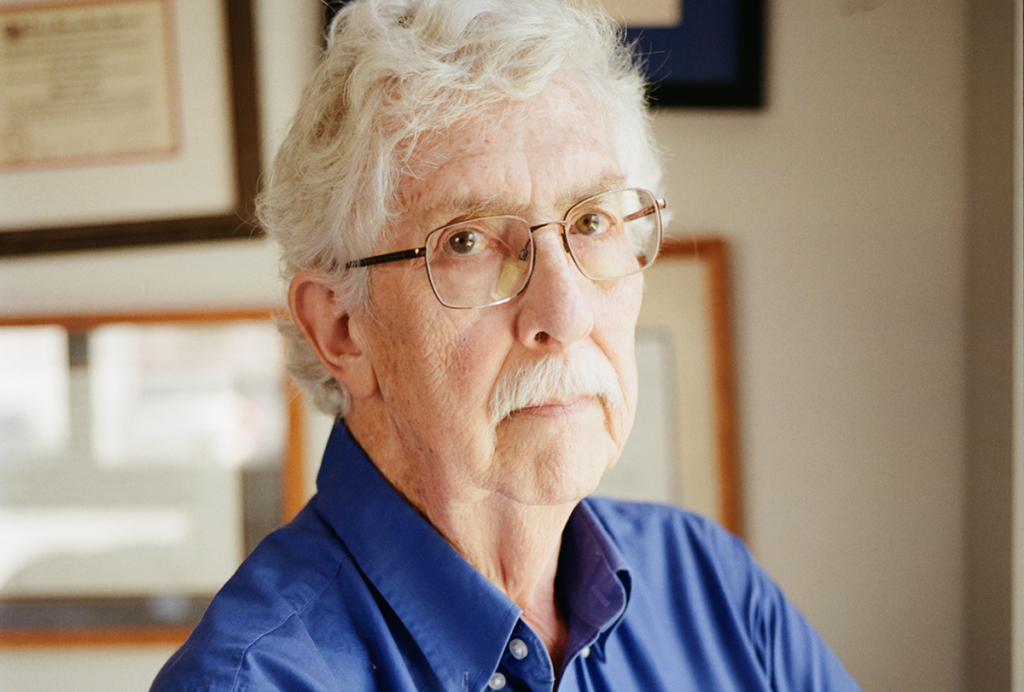
How pragmatism and passion drive Fred Volkmar—even after retirement
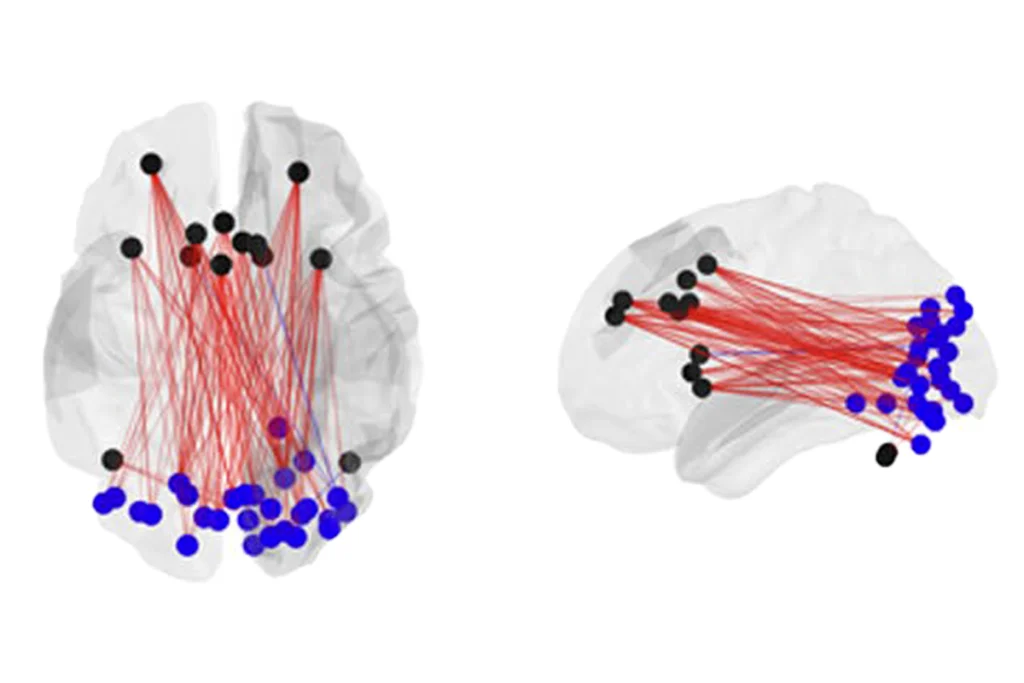
Altered translation in SYNGAP1-deficient mice; and more
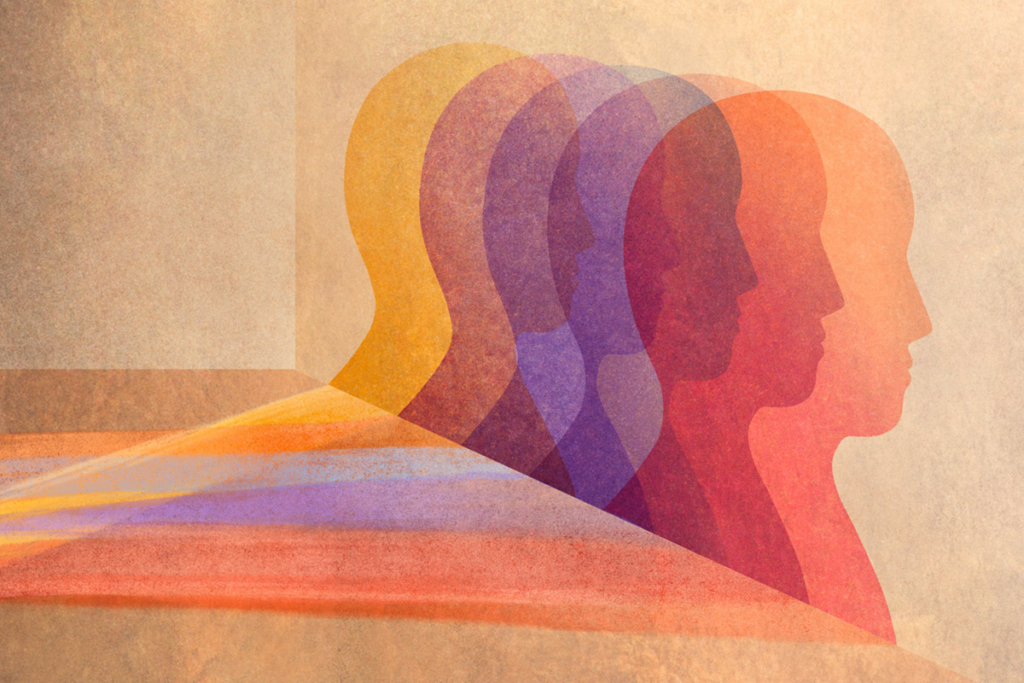
CDC autism prevalence numbers warrant attention—but not in the way RFK Jr. proposes
Explore more from The Transmitter
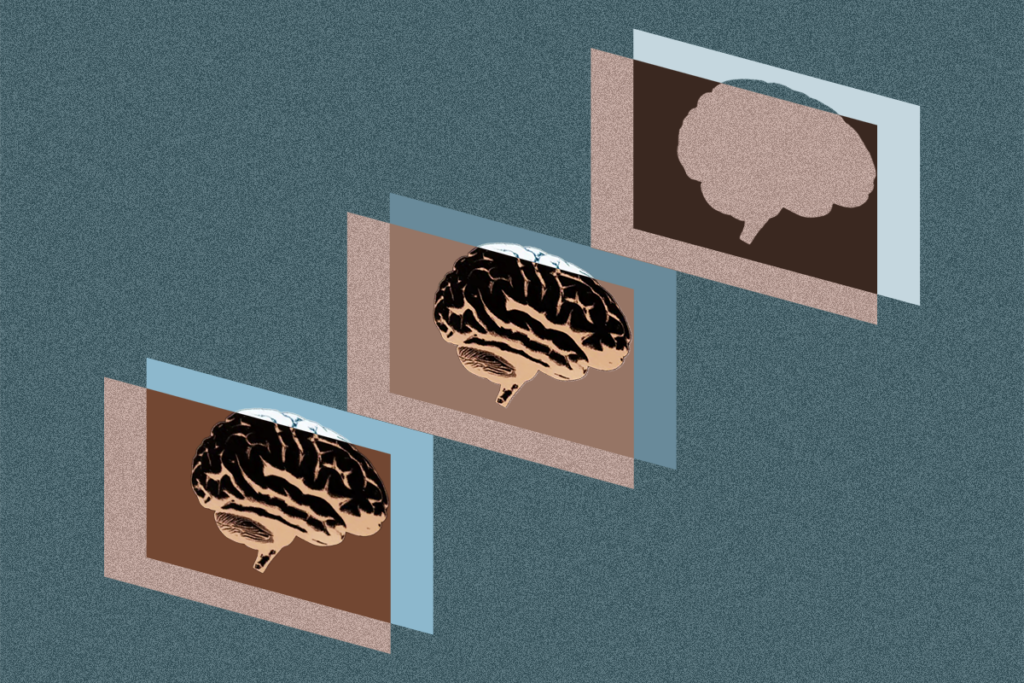