The 2003 paper linking neuroligins to autism
In 2003, Stephane Jamain and his colleagues reached a breakthrough by taking a candidate approach to the X chromosome, and linking members of the neuroligin protein family to autism.
Unraveling the etiology of autism has been one of the most puzzling challenges in medicine over the past decades. Although in his first description in 1943, Leo Kanner referred to autism as an innate disorder, prevailing views in the years that followed focused on environmental influences ― ranging from cold, unfit mothers to vaccines ― as the primary causative agents of the disorder.
By the 1990s, however, evidence from twin studies and increased susceptibility within families clearly indicated a strong genetic basis for autism spectrum disorders.
Over the past five to ten years, there have been major efforts towards uncovering the specific genetic alterations associated with autism. Chromosomal analysis and linkage studies have identified susceptibility loci on several chromosomes.
Interestingly, two of the loci reside on the X chromosome, which offers a potential explanation for the nearly four-fold greater incidence of autism in males compared with females.
However, until recently, only a few genes within any loci had been convincingly implicated in the disorder. A key question was whether disruptions in single genes could explain the complex phenotypes associated with the autism spectrum disorders, or whether multi-genetic contributions presented the major hindrance in the hunt for genes.
In 2003, Stephane Jamain and his colleagues reached a breakthrough in these genetic studies by taking a candidate approach focusing on genes in the autism-associated loci Xq13-21 and Xp22.31. Two genes in particular, NLGN3 and NLGN4 ― which encode members of the neuroligin protein family ― caught their attention.
Neuroligins are heterophilic cell adhesion molecules expressed in the brain, where they play critical roles in the organization of synapses. Jamain and colleagues hypothesized that disruptions in such genes lead to neuronal signaling defects that might underlie autism.
To test this hypothesis, they screened 36 pairs of affected siblings and 122 trios with autism or Asperger Syndrome for mutations in the coding regions of NLGN3 and NLGN4. In their Nature Genetics paper, they reported a missense mutation in NLGN3 (R451C) and a truncation in NLGN4 (396X) in affected siblings from two Swedish families.
Follow-up studies identified further mutations in NLGN4 in families with a history of autism or mental retardation2. However, to date no other patients with NLGN3 mutations have been reported. From all presently available studies, it appears that mutations in the neuroligin genes account for only a small percentage of autism cases.
Nonetheless, the discovery that apparently single-gene defects are associated with autism provided a new framework to begin understanding the pathophysiology of the disorder. Whatʼs more, it presented an opportunity to examine cellular abnormalities caused by such mutations in animal models.
Cellular studies and mouse models:
The next challenge in the field has been to determine the normal role of the neuroligin proteins at synapses and how the disease-associated mutations affect their function.
In 2003, Huda Zoghbi proposed the ‘synapse dysfunctionʼ hypothesis, which predicts that deficits seen in autism and related neurodevelopmental disorders, such as Rett Syndrome, might arise from aberrations in synaptic function3.
In support of this hypothesis, cell biological studies in neurons have demonstrated that the autism-associated mutations in NLGN3 and NLGN4 impair trafficking of the neuroligin proteins to the cell surface4,5 and, as a result, disrupt the function of the mutant neuroligin proteins at synapses.
To directly test how these mutations might affect synaptic signaling and behavior in vivo, different research teams have generated knock-in and knock-out mice that recapitulate the genetic defects identified in human patients6,7. The initial characterization of these mice suggests that they might be promising models for autism.
Notably, the mutant animals perform normally in an array of behavioral tests, including tests of learning and memory function. However, studies on both models reported aberrant social interactions, as the mutant animals appear to spend less time interacting with other mice compared with wild-type littermates.
The NLGN4 mutant mice show decreased vocalizations, mimicking the deficit in communication that is a hallmark of the human disorder. Although these initial results are promising, additional work will be required to confirm these conclusions.
A follow-up study on the NLGN3 mutant mice failed to uncover strong behavioral phenotypes, highlighting the importance of using standardized tests for behavioral analysis8.
On the cellular level, initial electrophysiological studies have found alterations in synaptic transmission in both NLGN3 and NLGN4 mutant mice.
These alterations could be due to aberrant functioning of individual synapses in the adult animals. Alternatively, loss of neuroligin function might result in the assembly of disorganized neuronal networks during development.
Given the broad expression of NLGN3 and NLGN4 in neurons throughout the brain, it is unclear why loss of neuroligin function would affect brain circuitry governing social behavior and communication, while leaving other networks apparently intact.
Last summer, Christopher Walsh and colleagues identified several homozygous deletions in individuals with autism that might offer some light into this conundrum9.
Remarkably, several of the genes within these deletions appear to be regulated by neuronal activity. Based on this correlation, the authors speculate that not synapse formation per se, but specifically activity-dependent synapse formation might be altered in autism and thereby prevent the normal tuning of neuronal circuits.
In only a few years, the study of neuroligin mutations has been a great step forward in advancing our understanding of autism. Although mutations in the NLGN genes are rare, subsequent studies have identified mutations in genes that act in the same signaling pathway as neuroligins, including neurexin-110,11,12 and SHANK313.
Although neuroligin mutations alone appear to result in an autistic phenotype, it remains likely that gene-gene and gene-environment interactions act in concert to increase the risk of developing autism.
One important future direction will be the combination of genetic models of disease with environmental insult to better understand the neurobiology underlying such cases of autism. Additionally, the synaptic signaling pathways and cellular defects defined in the genetic studies should provide a starting point for the design of therapeutic strategies.
References:
- Jamain S. et al. Nat. Genet. 34, 27-29 (2003) PubMed
- Laumonnier F. et al. Am. J. Hum. Genet. 74, 552-557 (2004) PubMed
- Zoghbi H.Y. Science 302, 826-830 (2003) PubMed
- Chih B. et al. Hum. Mol. Genet. 13, 1471-1477 (2004) PubMed
- Comoletti D. et al. J. Neurosci. 24, 4889-4893 (2004) PubMed
- Tabuchi K. et al. Science 318, 71-76 (2007) PubMed
- Jamain S. et al. Proc. Natl. Acad. Sci. USA 105, 1710-1715 (2008) PubMed
- Chadman K.K. et al. Autism Res. 1, 1-12 (2008) Abstract
- Morrow E.M. et al. Science 321, 218-223 (2008) PubMed
- Yan J. et al. Neurosci. Lett. 438, 368-370 (2008) PubMed
- Kim H.G. et al. Am. J. Hum. Genet. 82, 199-207 (2008) PubMed
- Autism Genome Project Consortium et al. Nat. Genet. 39, 319-328 (2007) PubMed
- Durand C.M. et al. Nat. Genet. 39, 25-27 (2007) PubMed
Recommended reading
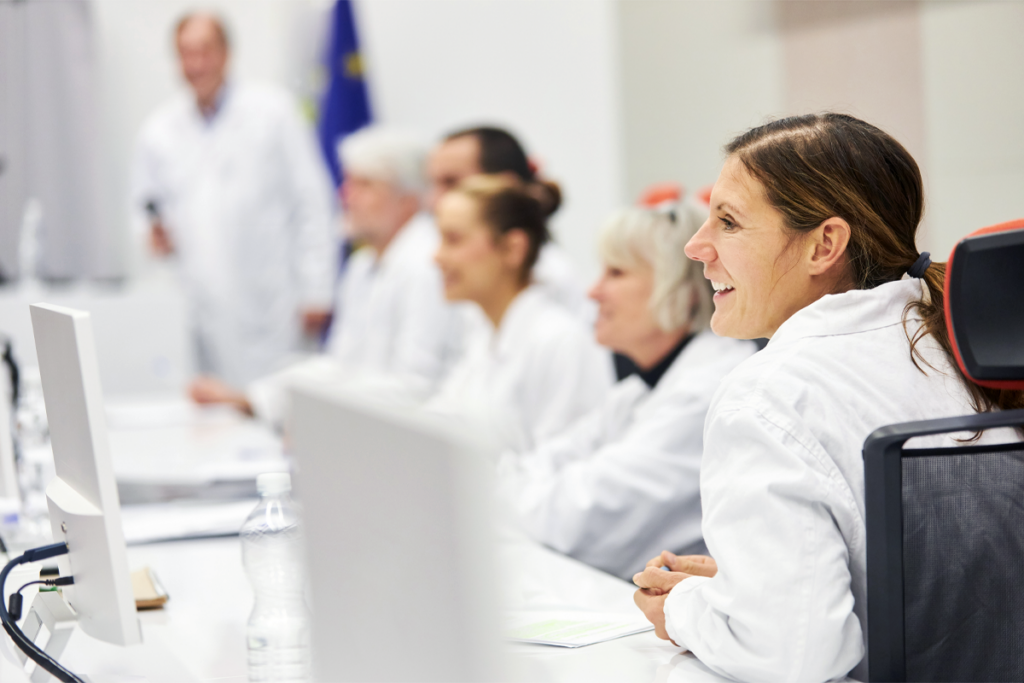
INSAR takes ‘intentional break’ from annual summer webinar series
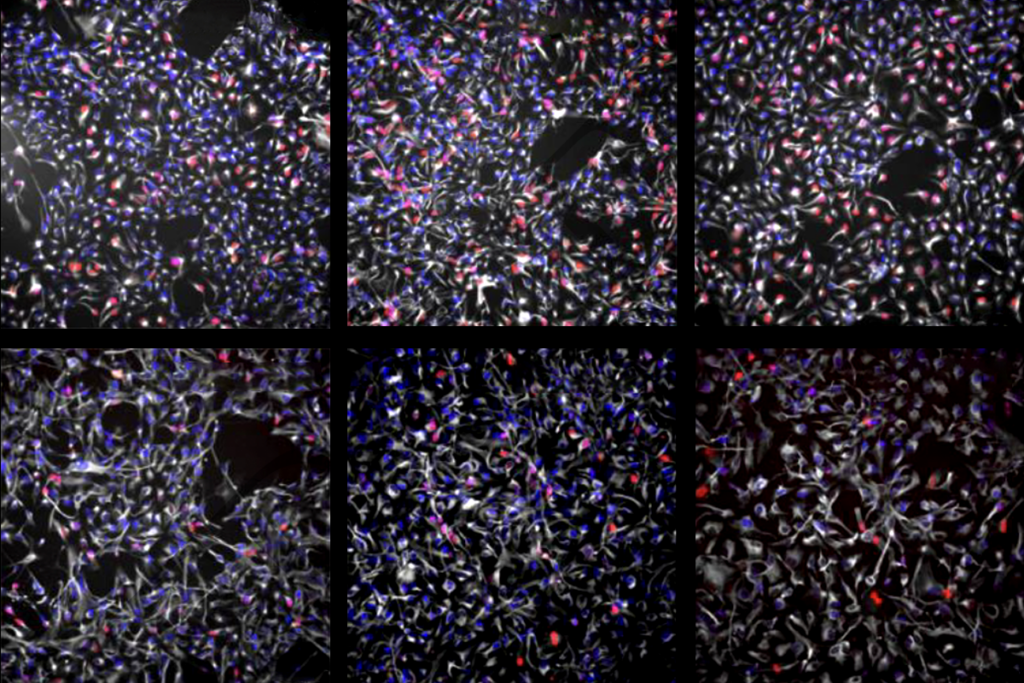
Dosage of X or Y chromosome relates to distinct outcomes; and more
Explore more from The Transmitter
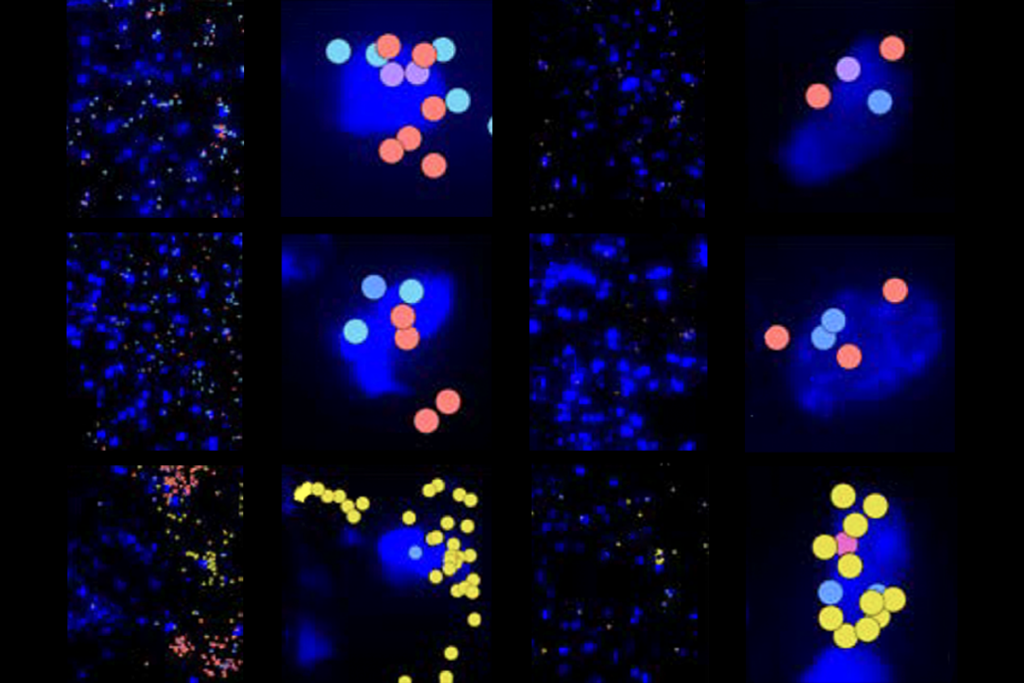
Machine learning spots neural progenitors in adult human brains
Xiao-Jing Wang outlines the future of theoretical neuroscience
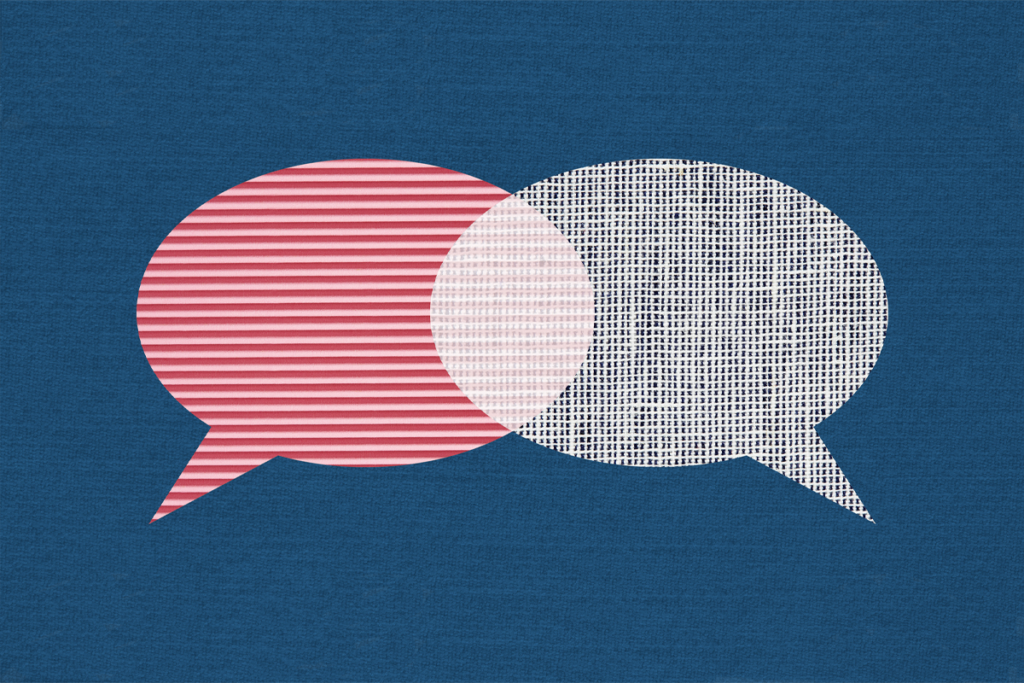