Escaping groupthink: What animals’ behavioral quirks reveal about the brain
Neuroscientists have long ignored the variability in animals’ behavioral responses in favor of studying differences across groups. But work on the brain differences that underlie that variability is beginning to pay off.
The mice were, for all intents and purposes, identical: They were all derived from the same inbred colony, making them genetically the same, and they were all reared in a shared environment. At first, they all behaved identically, too, in the 2019 study on alcohol consumption at Vanderbilt University—they quickly learned how to access the drinking spout and imbibed similar amounts of the intoxicating liquid.
But noticeable differences emerged several weeks later, after the mice were allowed to drink freely—hinting that they were not so identical after all. Given their druthers, a few animals consumed only a little alcohol; others drank more than average, as long as the alcohol wasn’t mixed with bitter-tasting quinine; and still others drank compulsively, regardless of the taste.
It may seem intuitive that the individual mice would diverge behaviorally, says study investigator Cody Siciliano, assistant professor of pharmacology at Vanderbilt. After all, even people who are identical twins are not exactly the same. But historically, most behavioral neuroscience experiments have been set up to minimize any chance of behavioral idiosyncrasies in favor of spotting group differences in response to various treatments, he says.
Any variability within a group—such as the disparate responses to alcohol among the mice—has tended to be seen as a nuisance, says Kay Tye, professor of neuroscience at the Salk Institute for Biological Studies, who led the 2019 alcohol consumption work.
Rather than dismissing that variability, Tye, Siciliano and their colleagues are exploring what individual variability can reveal about the brain, Tye says—with intriguing results. For example, a mouse’s brain activity during its first drink predicts its total alcohol consumption, they found in their study. The stronger the inhibition in cells that connect the prefrontal cortex and a midbrain region called the periaqueductal gray, the more likely a mouse is to drink compulsively. And an animal’s social rank—which the team could decode from other prefrontal cortex activity—also predicts those later drinking behaviors, according to preliminary data Tye shared at a workshop dedicated to individual differences, which she helped organize at the Computational and Systems Neuroscience (COSYNE) meeting in March.
Other researchers, too, have benefited from refocusing their efforts on individual variability, with recent discoveries of how the randomness by which circuits wire up contributes to a fly’s odor preferences, and how distinct patterns of brain activity underlie person-to-person differences in performance on a decision-making task.
As applied to systems neuroscience, this area of research is in early stages, and neuroscientists are approaching it in different ways, Siciliano says. But plenty of data already exist in researchers’ notebooks and hard drives, he adds. It’s just a matter of teasing out the differences worth studying.
Besides, there’s no point in pretending those differences within groups do not exist, he says. “Even if you try, you can’t get rid of the variability. It’s going to be there no matter what.”
T
he recent uptick in studying individual differences may stem from a shift in how neuroscientists design behavioral tasks, Siciliano says.More complex and naturalistic experiments—including open-ended tasks that have more than one solution—leave “a lot more room for individual differences to surface,” says Steve Chang, associate professor of psychology and neuroscience at Yale University. In tracking animals’ performance on these less-constrained tasks, aided by advanced machine-learning tools, researchers are interested in not just whether the task is completed but how the animals get there.
When marmosets, for example, have to pull a lever at the same time as a partner to get a reward, they change up their strategy depending on the partner’s sex and social rank, according to research Chang presented at the COSYNE workshop. And the cooperative strategies individual marmosets use seem to reflect differences in neuronal population dynamics in their dorsomedial prefrontal cortex.
Individuality even emerges within computational models when tasks become complex, according to unpublished work that Bing Wen Brunton, professor of biology at the University of Washington, presented at the workshop. Brunton and her colleagues trained artificial agents to use odor plumes wafting through a virtual arena to find a food source. When given a wind direction, the agents typically used similar strategies to reach their goal, the team found in a previous study. But when the agents had to infer the wind direction from other cues, they each developed their own idiosyncratic strategies. One agent, for example, made wide, systematic loops, which helped it locate the food source even if the wind changed direction frequently, Brunton says; others relied on sharp turns.
“If you look closely, they’re good at different parts of the task,” she says. “And so isn’t that what we mean by individuality, to some extent?”
But individuality can also emerge in simple tasks if researchers know where to look, says Benjamin de Bivort, professor of organismic and evolutionary biology at Harvard University, who studies genetically identical fruit flies. He has designed more than a dozen assays that measure about 200 different fly behaviors—including temperature response, spontaneous locomotion and how quickly they learn—and with each, he says, “we haven’t yet really found a behavior that we can convince ourselves has no individuality to it.”
One assay tests a fly’s odor preference. Flies display idiosyncratic preferences for one unpleasant odor over another, de Bivort and his colleagues found in a 2019 study when they presented the two scents at either end of an enclosed tunnel and observed the flies’ behavior.
Their preference, it turns out, correlates with the activity of projection neurons in the olfactory circuit: Calcium responses in these neurons differ for flies with different behavioral responses, de Bivort reported in a March paper. Stronger synaptic densities between olfactory receptor neurons and projection neurons predict a preference for one odor over another, imaging of a presynaptic protein in the axon terminals of olfactory receptor neurons revealed. And differences in synaptic strengths between the two cell types, which could arise by chance during development, can generate the neuronal activity associated with different odor preferences, modeling of the circuit using the fly connectome further demonstrated.
“The sort of big hypothesis behind our work is that stochastic variation in development drives microscopic differences in circuits that matter for behavior and drive individuality,” de Bivort says. And because the fly connectome is so well mapped, he and his team have been able to pinpoint the specific type of synaptic connection that shapes this particular behavior.
I
dentifying this sort of “locus of individuality” becomes more challenging in animals without an available connectome, de Bivort says. “What is different from one animal’s brain to the next that accounts for the differences in their behavior bias?” he asks. “Most of the tools of behavioral neuroscience aren’t particularly amenable to this question.”For their part, Tye and Chang have turned to past research to guide their investigations of circuits that seem to contribute to the behaviors they study. But even with a wiring map, figuring out the source of individual variation may be challenging, Chang says. Some behaviors could arise because of different levels of circulating neuromodulators, or even based on how neural computations are implemented, he says. “There’s a pretty interesting research direction there.”
Another challenge to this line of research is how to properly analyze results from the less controlled, more ecologically valid experiments that produce a variable behavior of interest, Tye says. If five mice are interacting in a cage, for example, “not every mouse is going to do every behavior—certainly not the same amount. And that creates a lot of problems for statistics and analyzing data that we as a field have to evolve past,” she says.
Other researchers are grappling with how to power their studies when they need to make multiple measurements in individual animals, says William Menegas, senior research scientist at the Broad Institute, who studies how well SHANK3 marmoset models recapitulate a genetic form of autism in people.
Half of Menegas’ SHANK3 marmosets become frightened when they view a picture of an unfamiliar animal, and the other half do not respond—both atypical responses, he says. On top of that, though, each monkey has its own unique behavioral performance on other assays, such as tests of cognition, anxiety and sociability.
Analyzing all of those behaviors, which are important for validating an autism model, becomes statistically complicated, Menegas says. “There’s more axes, more degrees of freedom. You have to basically divide your p-value by the number of those tests that you’ve done.” All of this also requires more animals and more data—which may be feasible for studies of flies and mice but less so for monkeys and people, he says.
Still, this variability is worth studying, Chang says. For one, understanding these behavioral differences can not only help explain how the brain gives rise to variable behaviors—it can also help improve clinical treatments. A treatment that may seem effective for one group can fail during a trial if the people within the group have high variability, he says. In that way, flattening results across participants “has kind of backfired in the field for a while.”
Looking at group averages can help get a study started, he says. But identifying the cracks within a group—what is not shared—can perhaps reveal more about how the brain works, Chang says. “That potential promise is clearly there.”
Recommended reading
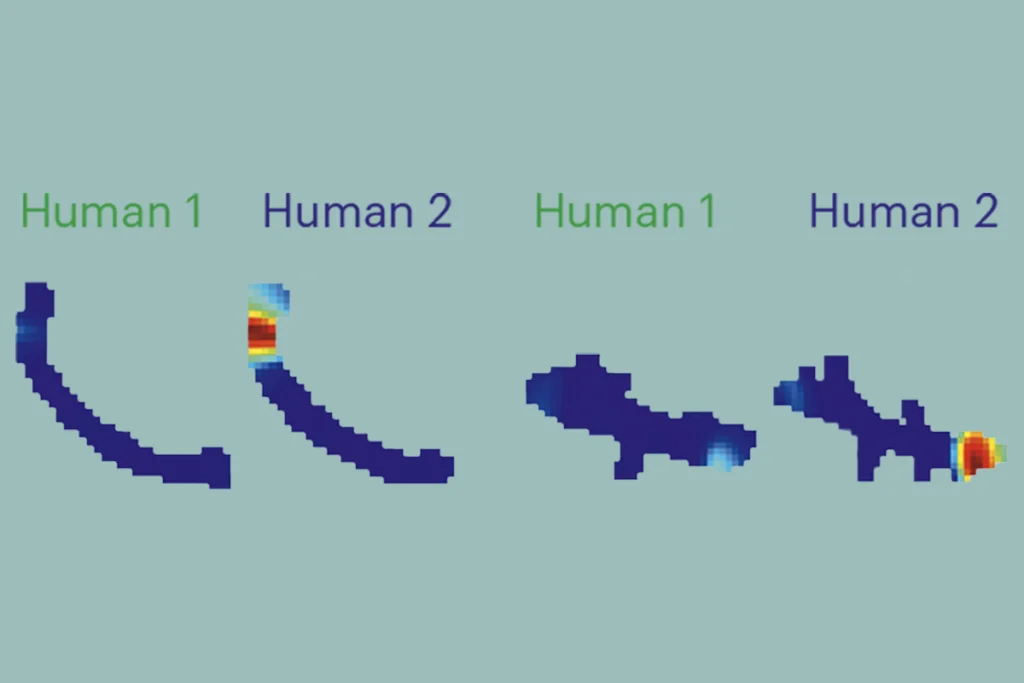
Egyptian fruit bats’ neural patterns represent different experimenters
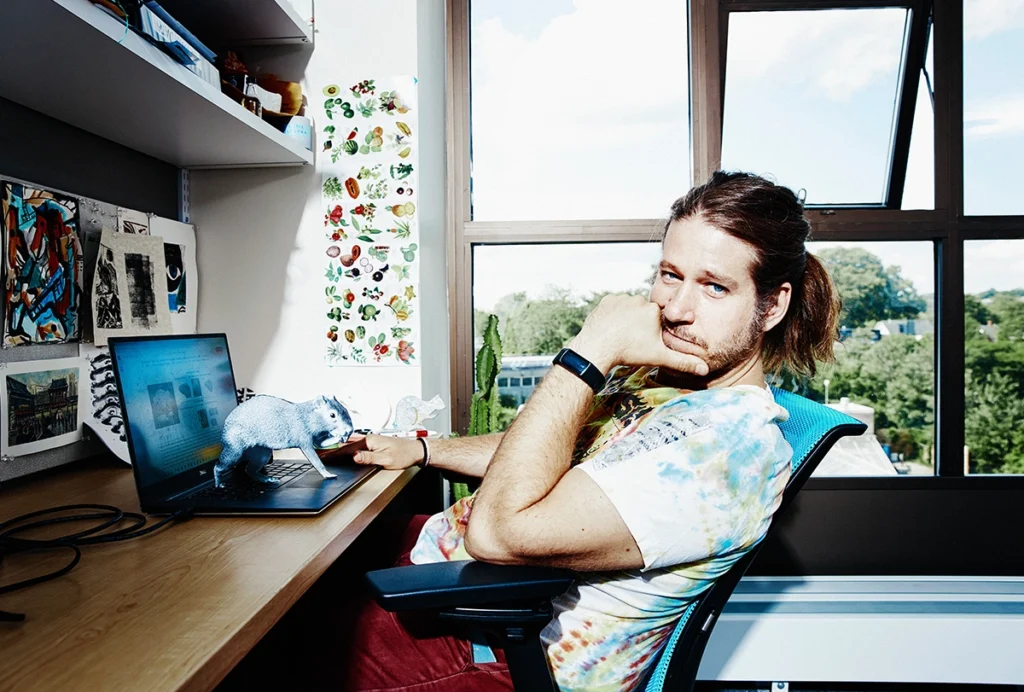
Improvising to study brains in the wild: Q&A with Nacho Sanguinetti-Scheck
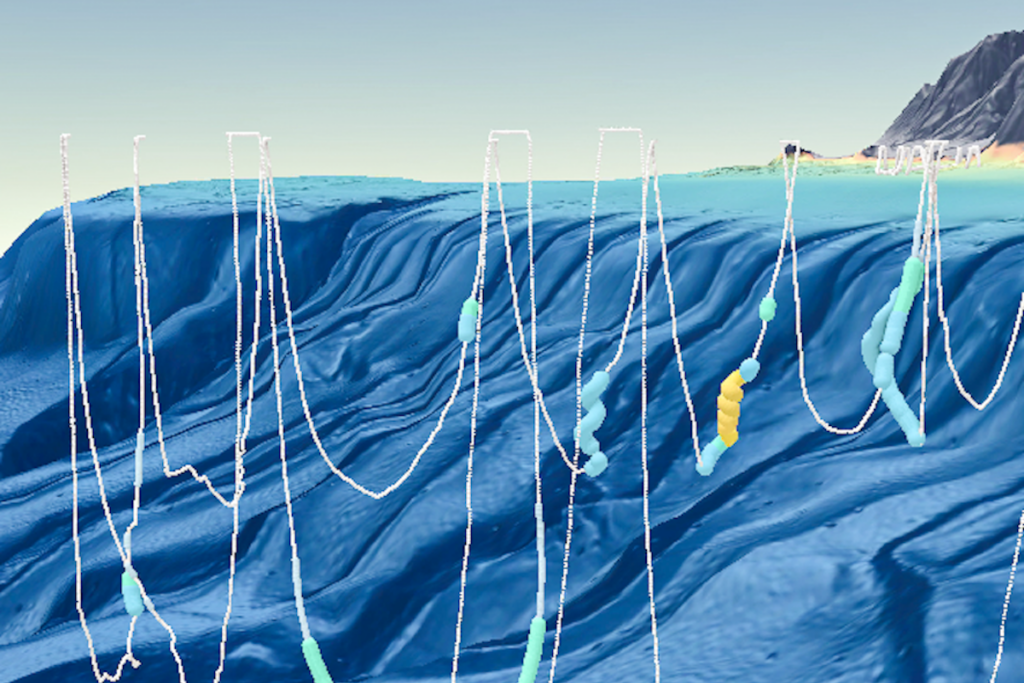
Wild and free: Understanding animal behavior beyond the lab
Explore more from The Transmitter
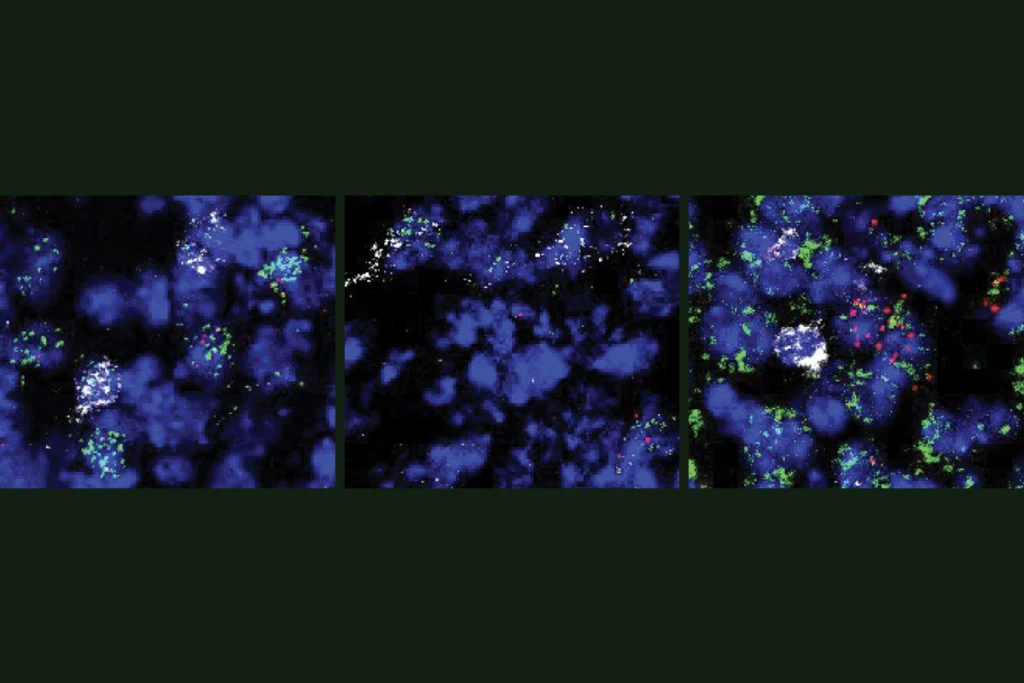
Novel neurons upend ‘yin-yang’ model of hunger, satiety in brain
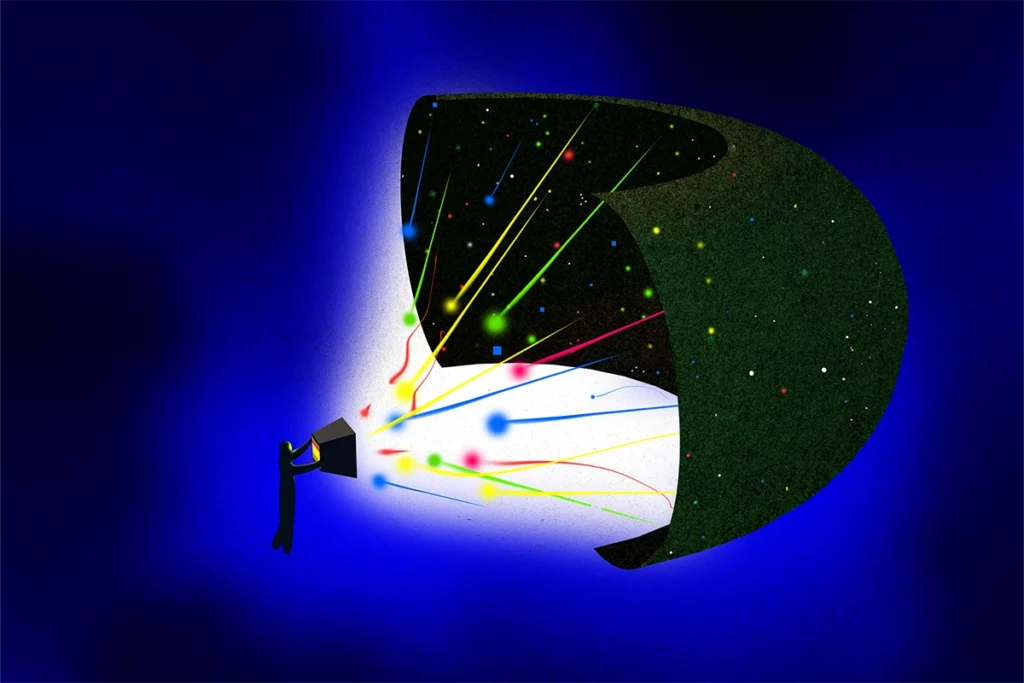
Imagining the ultimate systems neuroscience paper
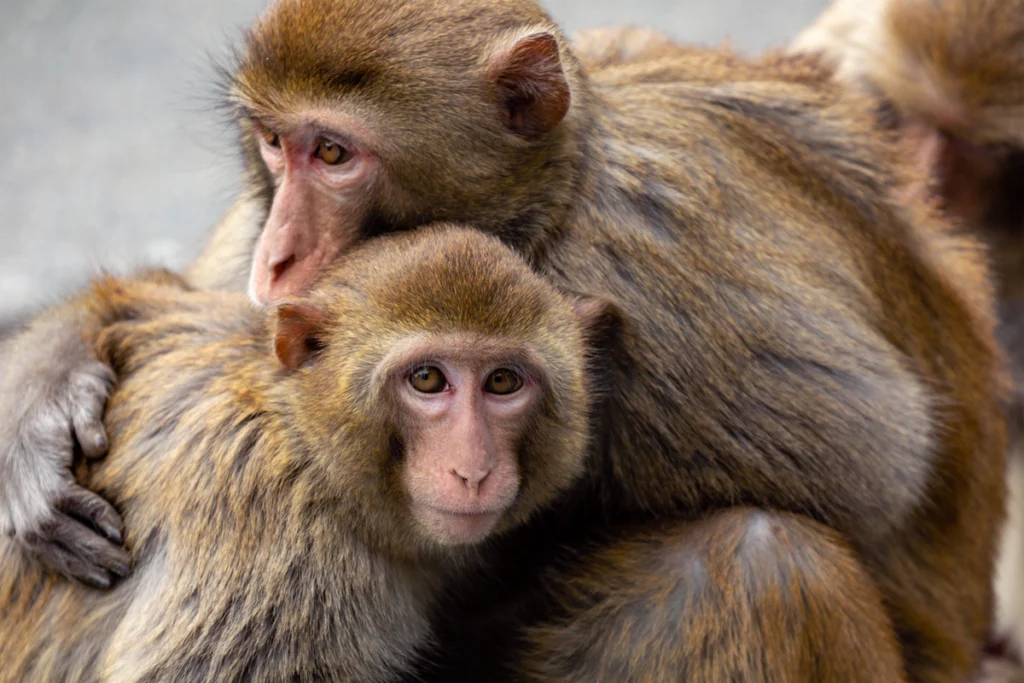