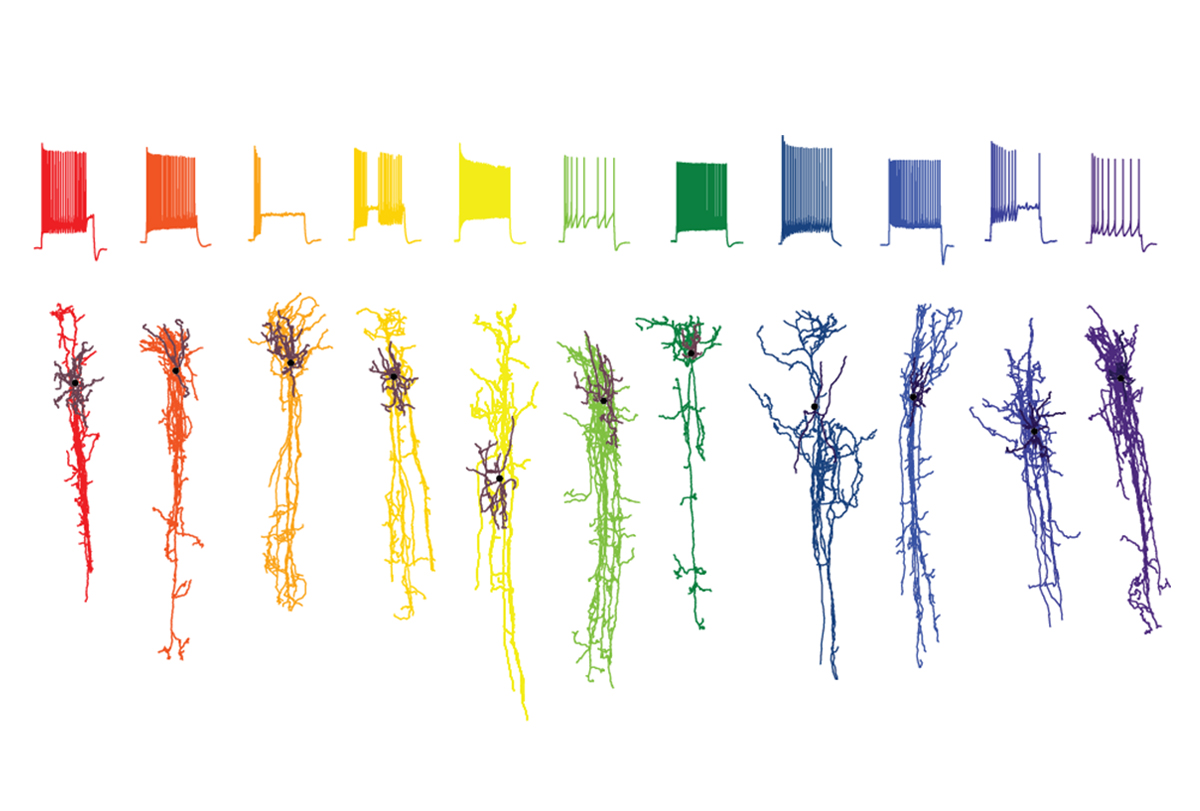
Vast diversity of human brain cell types revealed in trove of new datasets
The collection offers a glimpse into differences in cell composition — across people and brain regions — that may shape neural function.
New catalogs detail thousands of cell types that populate the human brain and that of our closest primate relatives. The findings appear in 21 papers published today in Science, Science Advances and Science Translational Medicine.
“It’s a huge, huge enterprise,” says Joshua Sanes, professor of molecular and cellular biology at Harvard University, who was not involved in the work. “It really is what translational neuroscience and systems neuroscience, as well as molecular neuroscience, will be building on for the next 10, 20 years.”
The new work used single-cell molecular approaches to identify more than 3,000 distinct neuronal and non-neuronal cell types within the developing and the adult human brain. Individual studies also compare cell composition across samples from 75 people and eight cortical regions, and across humans and four non-human primate species.
This effort represents a step toward a single-cell atlas of the human brain and begins to provide information about “what might make us uniquely human at the cellular level,” says Andrea Beckel-Mitchener, deputy director of the U.S. National Institutes of Health’s BRAIN Initiative, which funded the project.
The resulting datasets, which are available online, are the product of five years of research by more than 250 researchers who make up the BRAIN Initiative Cell Census Network (BICCN), a project that launched in 2017 to catalog mammalian brain cell types. In 2021, the BICCN team unveiled detailed atlases of the motor cortex in human, macaque and marmoset brains. And earlier this year, BICCN scientists posted preprints that establish the most complete cell atlases of the mouse brain to date.
The datasets provide researchers with benchmarks for typical cell composition across humans, non-human primates and other mammals, says Ed Lein, senior investigator at the Allen Institute for Brain Science in Seattle, Washington, and a researcher on multiple papers in today’s collection. And in the future, they could help scientists select the best model organisms for a given study — and even identify cells implicated in disease, he says.
T
Some scientists have long questioned whether single-cell transcriptomics could distinguish cells in ways that are as useful as morphology or physiology have been for decades, Sanes says — “it’s biology; it’s complicated.” But the new work suggests that identifying cells by their molecular components offers a complementary, if not always overlapping, way of categorizing cells throughout the brain, he says.
There is utility in describing the cells’ gene expression, agrees Jason Shepherd, associate professor of neurobiology at the University of Utah in Salt Lake City, who was not involved in the new work. “The subsequent interpretation of what that means is where it starts to get a little muddy.”
Even researchers involved in the BICCN were initially uncertain about whether their single-cell techniques, which were originally used in animals, would translate to people, particularly at a large scale. Anything from difficulty in handling the samples of human tissue to unforeseen species differences could have thwarted the work, Lein says.
But the new work confirms that single-cell methods can reveal new insights about the cell composition of our own species, Lein says. “It’s really quite remarkable. The diversity that we all thought was there, really is there.” That diversity is highest in the midbrain and hindbrain, the new work shows —areas that have been studied less extensively than the cerebrum.
Throughout the brain, cell composition relates to function, the new work suggests. The same 24 major cell types appear consistently across all eight areas of the cerebral cortex that Lein and his colleagues sampled in one of the new studies, but these cells’ proportion changes from region to region. One brain region that stands out for its unusual composition is the primary visual cortex, which the new work shows has distinct subtypes of excitatory and inhibitory cells. That likely reflects the fact that the primate brain is “wildly specialized” for visual inputs, Lein says.
Different people also tend to share all the same brain cell types but vary in how those cells — particularly excitatory neurons and glia — express genes, according to another study, which examined live tissue samples collected from 75 people as they underwent surgery to treat various brain conditions. Most of that variation is not explained by demographic factors such as age or sex but instead may stem from things such as life experience, says study investigator Trygve Bakken, assistant investigator at the Allen Institute for Brain Science.
Another new study revealed that children with early-life brain inflammation have changes to gene expression in two types of inhibitory neurons within their cerebellum. Many of the affected genes have been linked to autism, pointing to a potential place of convergence for some forms of the condition.
Some of the new papers also document cell-type composition across non-human primates, including macaque monkeys and marmosets. These studies, coupled with the BICCN’s mouse atlases, offer a way to assess which cell types are shared across evolution and which are specific to humans — findings that can help researchers select the best model organisms for a given study.
“This really opens the door to ask, ‘Can we translate findings from model organisms to humans?’” Bakken says.
Other studies, which document how brain cells change throughout the early stages of development, can help guide researchers who seek to model human development using organoids, Lein says.
T
Moving forward, the network aims to fill out the contours of the prototype atlas. Last year, it transitioned to its next phase, called the BRAIN Initiative Cell Atlas Network. “We’re ramping up to hundreds of donors across a much broader collection of ages and ancestries,” Beckel-Mitchener says.
Ultimately, the goal is that these atlases will provide “a good understanding of what the normal brain actually looks like,” Lein says. When used as a reference, the atlases can serve as a basis for understanding which cells are affected in disease, and when. That would enable researchers to ask “questions that are much more directed and much more data driven” when deciding which brain areas to study for a given condition, says Tomasz Nowakowski, associate professor of anatomy, psychiatry and neurological surgery at the University of California, San Francisco, who led one of the studies published today.
“There’s a lot of work to do,” says Seth Ament, associate professor of psychiatry at the University of Maryland School of Medicine in Baltimore, who worked on the study of inflammation in the cerebellum. “But that’s the mark of any important project, right?”
Recommended reading
Expanding set of viral tools targets almost any brain cell type
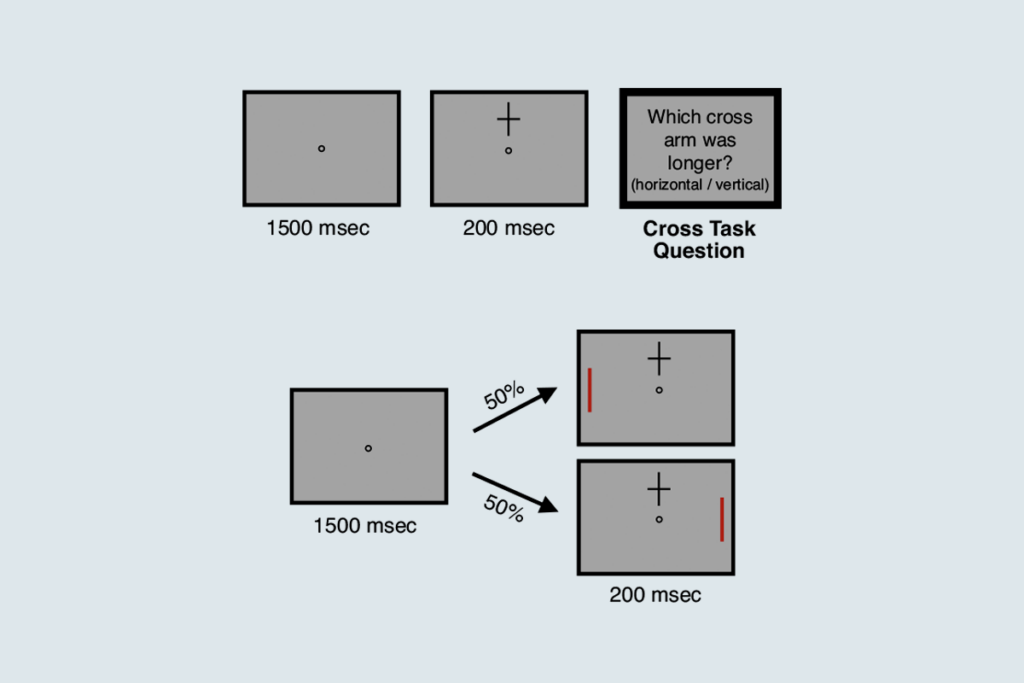
Attention not necessary for visual awareness, large study suggests
Explore more from The Transmitter
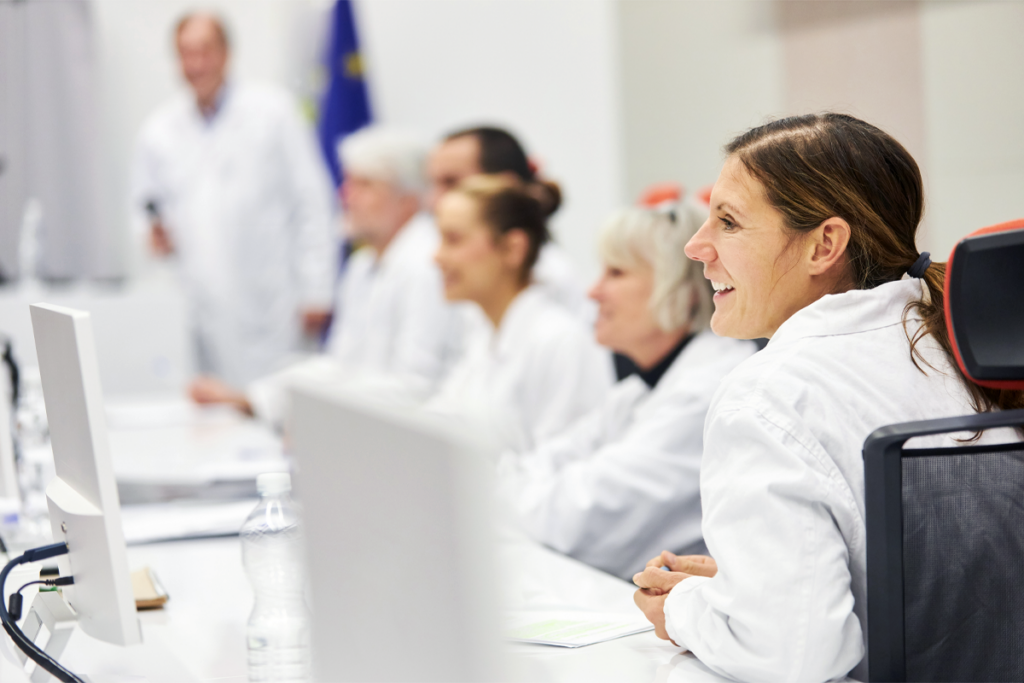
INSAR takes ‘intentional break’ from annual summer webinar series
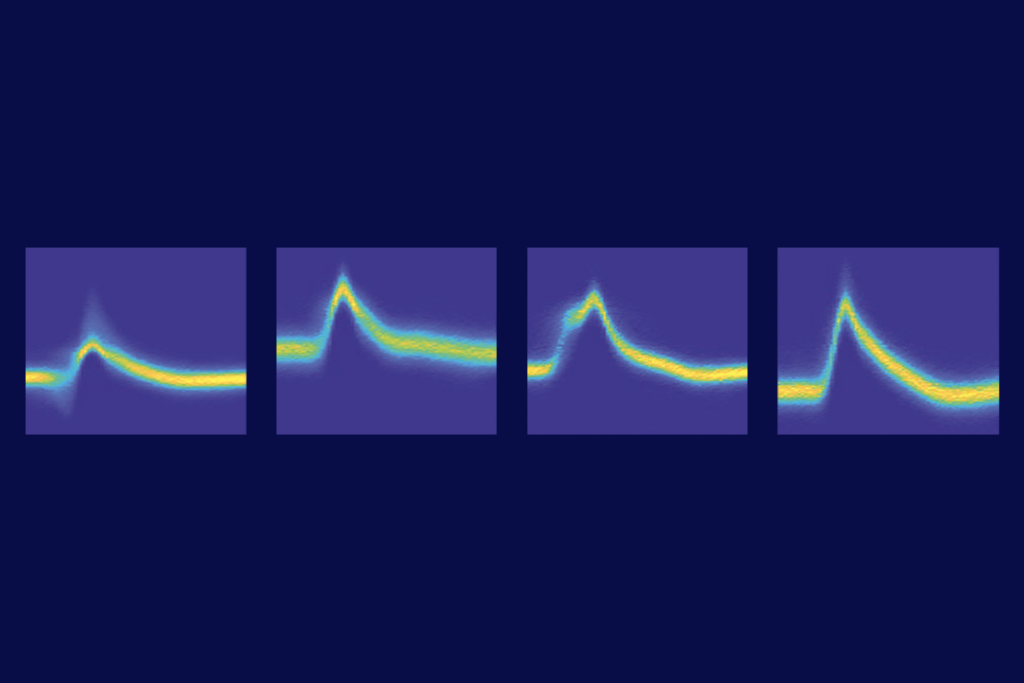