Exploring enigmatic links between mitochondria and autism
Mitochondrial deficits may account for the range of symptoms and neurological deficits seen in autism and explain why it preferentially affects boys, says Douglas Wallace.

Power source: Individuals with autism have mutations in a number of genes that affect the functioning of mitochondria, the energy source of the cell.
The most overt symptoms of autism are neurological, and as a result much of the research on this disorder has focused on the brain. But there is substantial evidence suggesting that a significant proportion of people with autism also have problems with other organs, including the intestines, muscle and the immune system.
It is possible that the underlying defect that causes a significant proportion of autism cases is present throughout the body, but preferentially affects the brain. This would be characteristic of disorders resulting from mild mitochondrial dysfunction1.
The mitochondria are located outside of the nucleus in the cytoplasm of the cell. These cellular power plants produce most of the cell’s energy by burning the calories in the food we eat, using the oxygen that we breathe.
A portion of the energy generated by the mitochondria is converted to adenosine triphosphate (ATP), which stores the energy in a chemical form that can then be transported to areas of the cell where energy is needed. The stored energy is released by hydrolysis of ATP into adenosine diphosphate (ADP) and phosphate1.
Unique among cellular organelles, the mitochondria have their own DNA (mtDNA). mtDNA codes for the key proteins involved in energy production — the wiring diagram of the mitochondrial energy production system, called oxidative phosphorylation (OXPHOS). However, nuclear DNA (nDNA) codes for between 1,000 and 2,000 genes that are required for the assembly, structure and function of a mitochondrion.
If autism is the result of mild mitochondrial defects, then the mitochondrial defects in autism are likely to be too subtle to be detected by the current relatively insensitive mitochondrial assays. Even so, some individuals with autism show signs of mitochondrial dysfunction.
Some individuals with the disorder have been found to manifest metabolic deficits related to mitochondria, such as alterations in ATP, ADP, phosphate, and phosphocreatine levels detected by P31 magnetic resonance spectroscopy (MRS), defects in mitochondrial OXPHOS enzymes in muscle, brain or lymphoblastoid cells, and mutations in the mitochondrial genes in either nDNA or mtDNA2-7.
Mitochondrial energy defects can result from any one of a myriad of alterations in either mtDNA- or nDNA-coded mitochondrial genes. Whereas nDNA is inherited from both the mother and the father, with each child inheriting two copies of each nDNA gene, mtDNA is inherited exclusively from the mother and each cell contains thousands of copies.
Mitochondrial energy defects may result from alterations in one or both copies of an nDNA mitochondrial gene, a variant present in different proportions of the mtDNA genes, faulty interactions between the nDNA and mtDNA genes or environmental factors that inhibit OXPHOS. Overall, the inheritance of mitochondrial defects can be complex.
Energy loss:
The brain accounts for only two percent of the body’s weight, but it consumes 20 percent of the oxygen we breathe. It is by far the organ most reliant on mitochondrial energy production for normal function. Subtle changes in mitochondrial energetics can result in neurological symptoms. Since researchers reported the first mtDNA mutations 24 years ago8 9 10, a wide variety of neurological diseases have been linked to mitochondrial genetic defects.
The mildest mitochondrial defects only affect the central nervous system. However, as the severity of the energetic defect increases, other organs begin to be affected. These include the heart, muscle (including intestinal muscles), hearing and peripheral nerves, the immune system and hormonal systems.
The acquisition of symptoms elicited by progressive mitochondrial energy decline is analogous to a metropolitan brownout, with electrical systems failing sequentially, based on their minimal electrical energy requirements, as the line voltage declines.
Because autism preferentially affects the brain, much interest has been focused on defects in the formation, structure and maintenance of synapses, the junctions between neurons11. However, synapse function also requires high mitochondrial energy production, making mitochondrial function and dynamics critical for generating and maintaining synapses and dendritic spines, the signal-receiving branches of neurons12 13 14. Mild mitochondrial defects may also result in synaptic dysfunction and, thus, autism.
For reasons not yet entirely understood, but probably related to hormonal differences, males are more sensitive to mild mitochondrial energy deficits than are females. This phenomenon was first observed for Leber hereditary optic neuropathy, the mildest disease associated with mtDNA mutations.
This disorder is three times more likely to affect males than females15. If autism also results from mild mitochondrial energy defects, this may account for the four-fold increased risk of autism for boys compared with girls16.
In addition to providing most of the cell’s energy, mitochondria also regulate cellular calcium levels, generate most of a cell’s reactive oxygen species (ROS) — frequently called oxygen free radicals — and regulate cell death. Normal mitochondria produce low levels of ROS, which are essential for cell signaling, but defective mitochondria can produce excessive ROS. These overwhelm normal ROS degradation systems and damage the cell and its mitochondria.
If the resulting oxidative damage becomes too severe, the cell activates a self-destruct system known as apoptosis. This removes the damaged cell before it can compromise the function of the rest of the tissue. In the brain, where the cells store and retrieve information by active neuronal communication, malfunctioning neurons may erode the quality of the brain’s information system.
Genetic alterations:
Researchers have invested extensive efforts in searching for alterations in nDNA genes that are associated with autism. One type of nDNA genetic alteration that is increased in autism is the number of copy number variants (CNVs). CNVs involve the deletion or duplication of a region of the nDNA within a chromosome. On average, people with autism have more CNVs than do unaffected individuals.
CNVs encompassing certain genes have been found to recur in autism, and some of these may act by affecting mitochondrial energy production. For example, PARK2, which is associated with Parkinson’s disease and autism17 18, is involved in mitochondrial quality control19. Mutations in UBE3A, which are linked to autism and the related Angelman syndrome, result in mitochondrial dysfunction in the hippocampus20.
Postmortem autism brains have found to contain elevated calcium levels21. Timothy syndrome, a syndromic form of autism, is caused by a mutation in the cell membrane calcium transporter gene CACNA1C22, and mutations in the related CACNA1F gene have also been found in individuals with autism23.
Mitochondria actively take up calcium, and its levels directly regulate mitochondrial energy production. Also, high cytosolic levels of calcium activate a mitochondrial transporter that regulates the flow of energy substrate into the mitochondrion. Excessive cytosolic calcium can enhance the import of energy substrate and its processing within the mitochondrion, ultimately overloading mitochondrial OXPHOS.
This results in an increase in mitochondrial ROS production, which may ultimately cause the mitochondria to initiate cell death by apoptosis. This is analogous to putting too much wood in a fireplace, which only results in generating excessive toxic smoke.
The excessive flow of calcium into the cell and the mitochondrion can result from faulty transport of calcium across the outer cellular (plasma) membrane, damage to the plasma membrane, or the release of calcium stored with cell compartments into the cytoplasm.
The excitatory neuronal transmitter glutamate opens calcium channels in the neuronal plasma membrane. Excessive glutamate stimulation can also result in excessive intracellular calcium and neuronal death.
Detailed analyses:
To test the link between mitochondrial dysfunction and autism, we performed a detailed analysis of CNVs in a well-characterized group of autism families. We confirmed that CNVs are more common in individuals with autism than in controls. Also, we found that many of these CNVs disrupt nuclear genes associated with mitochondrial functions6.
The most common CNV larger than 100 kilobases is at the 15q11-13.3 chromosomal region, which encompasses UBE3A. Other large CNVs have been found to encompass genes for subunits of respiratory complex I (NDUFA4and NDUFA10) and complex IV (COX5A), as well as genes for OXPHOS enzyme cofactors, metabolic enzymes, metabolite transport and mitochondrial biogenesis.
Two siblings with autism have a rearrangement in the 2p12p chromosomal region that deletes 27 genes, including the CACNA1C and CACNA2D4 calcium transporters. The rearrangement also duplicates 12 genes, including SNTG2, which is mutated in some individuals with autism.
To determine if loss of nuclear DNA genes might affect mitochondrial function, we analyzed the mitochondrial OXPHOS enzyme levels in two patients, one with only a few CNVs and another with multiple CNVs. In contrast to the individual with few CNVs, the individual with many CNVs has a clinically significant muscle mitochondrial OXPHOS defect.
Increasing biochemical and genetic evidence supports the hypothesis that a significant proportion of people with autism harbor mitochondrial energetic defects. The mitochondria have multiple functions and rely on 1,000 to 2,000 nuclear genes plus thousands of copies of the mtDNA to effectively provide energy. This means that a wide spectrum of possible minor genetic alterations in mitochondrial genes may be sufficient to impede brain function and cause autism.
Consistent with this hypothesis, mild mitochondrial defects preferentially affect males, who are overrepresented in autism. Therefore, the genetic and biochemical complexity of the mitochondrion readily encompasses the clinical variability and genetic complexity associated with autism.
Douglas C. Wallace is director of the Center for Mitochondrial and Epigenomic Medicine at the Children’s Hospital of Philadelphia.
References:
1: Wallace D.C. Annu. Rev. Genet. 39, 359-407 (2005) PubMed
2: Chauhan A. et al. J. Neurochem. 117, 209-220 (2011) PubMed
3: Giulivi C. et al. JAMA 304, 2389-2396 (2010) PubMed
4: Minshew N.J. et al. Biol. Psychiatry 33, 762-773 (1993) PubMed
5: Pastural E. et al. Prostaglandins Leukot. Essent. Fatty Acids 81, 253-264 (2009) PubMed
6: Smith M. et al. Biochim. Biophys. Acta. 1817, 1796-1802 (2012) PubMed
7: Weissman J.R. et al. PLoS One 3, e3815 (2008) PubMed
8: Shoffner J.M. Cell 61, 931-937 (1990) PubMed
9: Wallace D.C. et al. Science 242, 1427-1430 (1988) PubMed
10: Wallace D.C. et al. Cell 55, 601-610 (1988) PubMed
11: Pardo C.A. and C.G. Eberhart C.G. Brain Pathol. 17, 434-447 (2007) PubMed
12: Kang J.S. et al. Cell 132,137-148 (2008) PubMed
13: Liu Q.A. and H. Shio PLoS Genet. 4, e1000097 (2008) PubMed
14: Mattson M.P. and D. Liu Neuromolecular Med. 2, 215-231 (2002) Pubmed
15: Sadun A.A. et al. Curr. Treat. Options Neurol. 13, 109-117 (2008) PubMed
16: Filipek P.A. (2005) Medical Aspects of Autism. In F.R. Volkmar et al. (Eds.), Handbook of Autism and Pervasive Developmental Disorders (pp. 534-578) New York: John Wiley and Sons.
17: Gai X. et al. Mol. Psychiatry 17, 402-411 (2012) PubMed
18: Glessner J.T. et al. Nature 459, 569-573 (2009) PubMed
19: Narendra D. et al. J. Cell Biol. 183, 795-803 (2008) PubMed
20: Su H. et al. Neurosci. Lett. 487, 129-133 (2011) PubMed
21: Palmieri L. et al. Mol. Psychiatry 15,38-52 (2010) PubMed
22: Splawski I. et al. Proc. Natl. Acad. Sci. USA 102, 8089-8096 (2005) PubMed
23: Myers R.A. et al. PLoS Genet. 7, e1001318 (2011) PubMed
Recommended reading
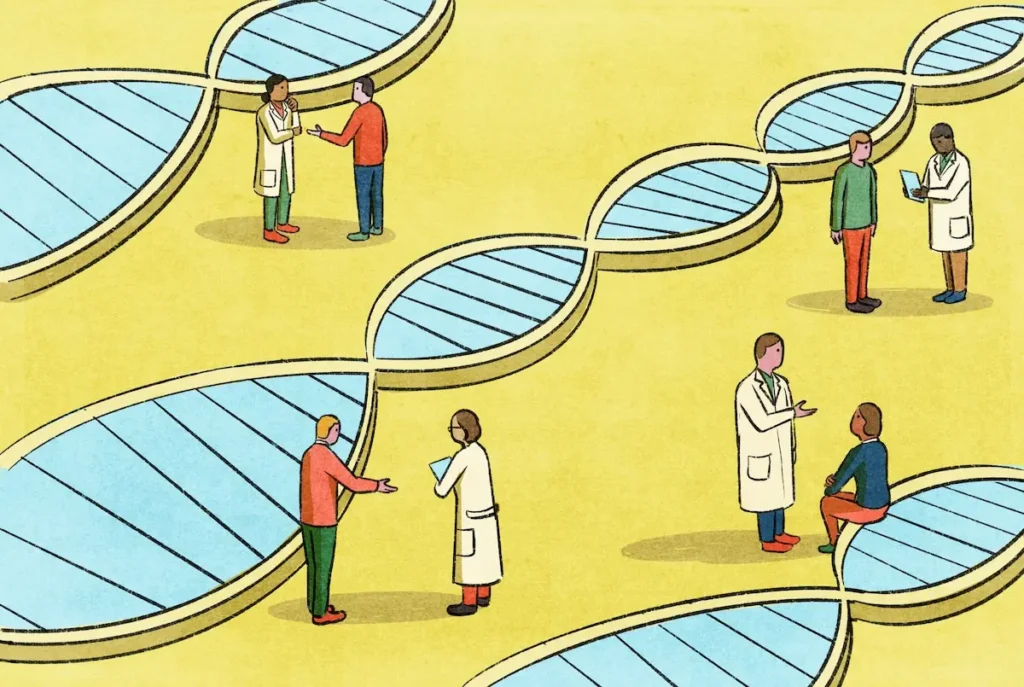
Building an autism research registry: Q&A with Tony Charman
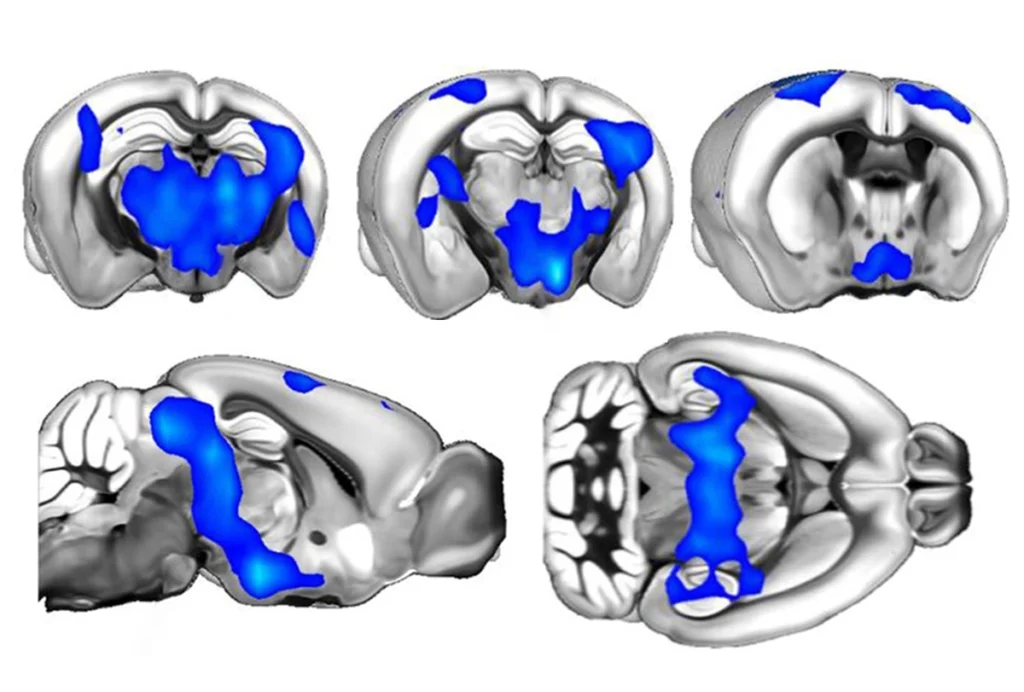
CNTNAP2 variants; trait trajectories; sensory reactivity
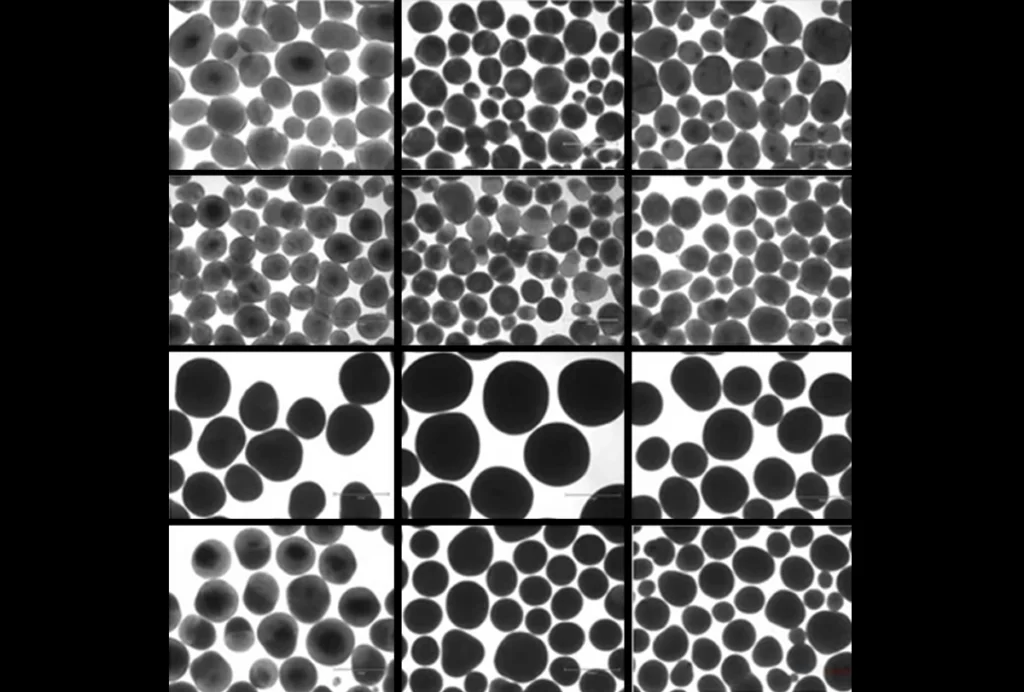
Brain organoid size matches intensity of social problems in autistic people
Explore more from The Transmitter
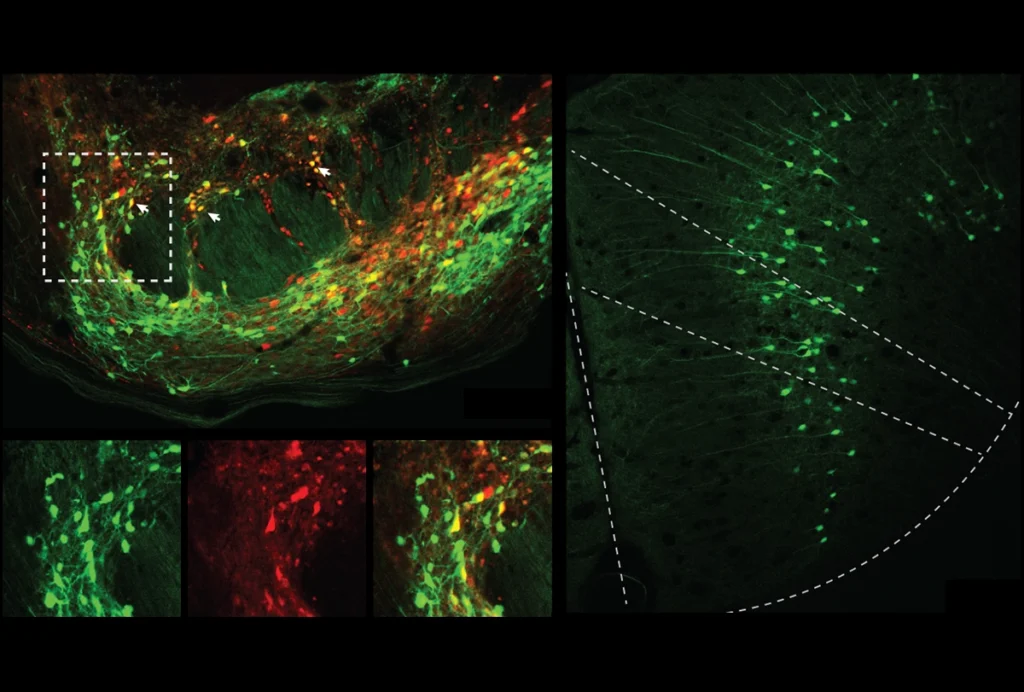
Cerebellar circuit may convert expected pain relief into real thing
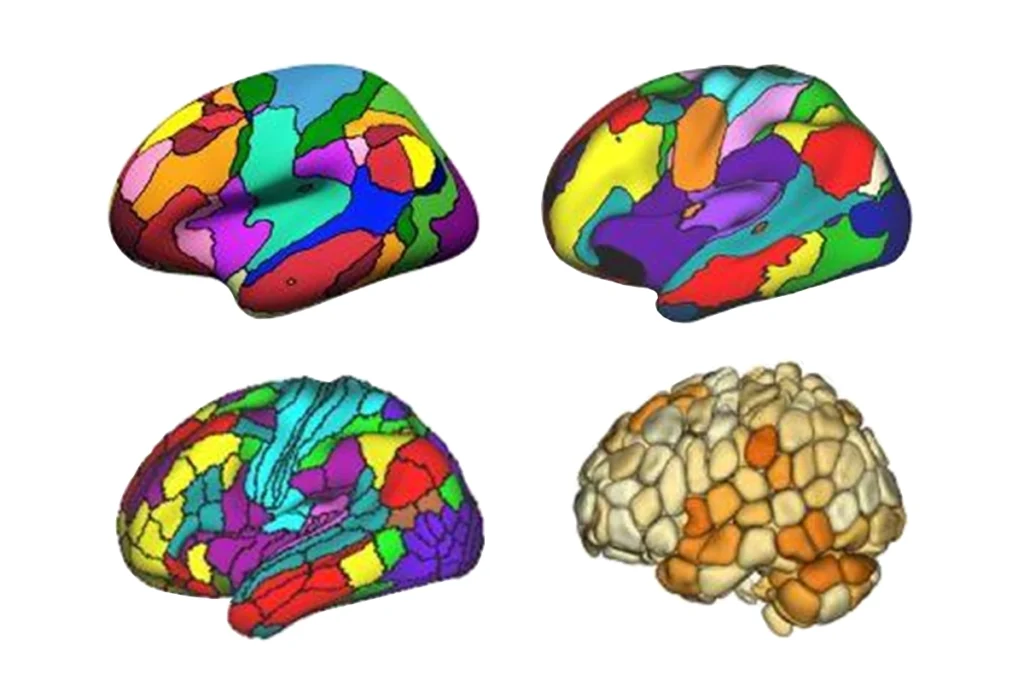